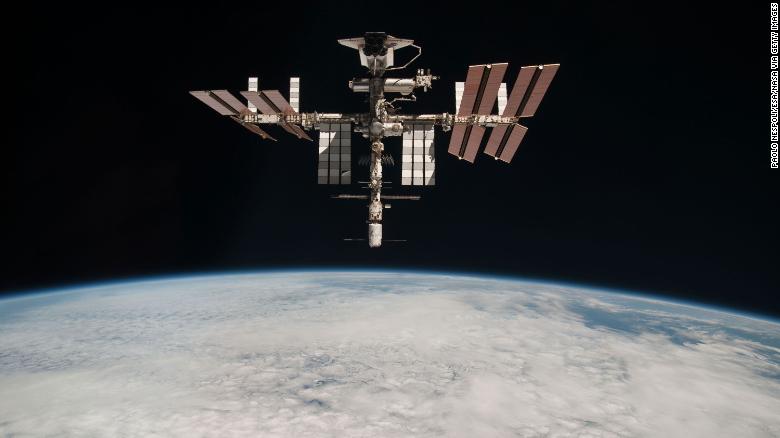
Telomeres in Space
By Katherine De Lange
In human imagination, life is commonly represented as threads woven together by fate, degrading as one ages. This representation might reveal some truth. Life expectancy and risk of disease is, in fact, associated with threads—our telomeres, which cap the ends of human chromosomes. Some researchers study telomeres to understand aging and disease; others strive for immortality by preventing telomere degradation. In recent years, the study of telomeres has entered space: researchers studied telomere shortening in astronauts to assess the limits of human exploration.
Telomere shortening is thought to be caused by the end replication problem. In DNA replication, the DNA double helix unwinds into 2 strands with opposite directionality. The DNA polymerase enzyme uses the original DNA strands as templates to add nucleotides, forming new DNA strands.
However, DNA polymerase can only add nucleotides in the 5’ to 3’ direction, so one of the strands is synthesized by the help of multiple primers. Primers provide the starting point for each small “okazaki fragment” in this lagging strand. After the completion of the fragments, primers are removed, and holes left behind by primers are fixed. At the very end of the DNA strand, though, a small gap is still present due to a lack of a strand or primer with a 3’ end before the site of replication.
Normally, genomic integrity is preserved due to the presence of telomeres, but after rounds of DNA replication, telomeres are degraded. If telomeres shorten significantly, cell replication machinery eats into genes essential for bodily functions. Telomerase, another enzyme, can restore telomeres, but its activity is insufficient to restore telomeres in normal somatic cells. Thus, aging (which implies that cells have undergone replication for many times already) leads to telomere attrition. In addition to a lack of telomere restoration, other factors such as oxidative stress, inflammation, as well as environmental factors may result in a net shortening of DNA caps.
A myriad of telomere research is taking place. One area of research, which examines external effects on telomeres, can identify harmful environments for human activity. One study by Luxton et al. (2020) investigates the effect of space travel on the telomeres of astronauts, which can be negatively regulated by factors such as space radiation.
Space radiation differs from radiation on earth. Galactic cosmic radiation strips away electrons from atoms moving close to the speed of light—and this is only one kind of radiation astronauts are exposed to. Luxton et al. (2020) assessed telomere length dynamics in three unrelated astronauts before, during, and after their six month or one year mission in space. Though it may seem like telomeres would steadily shorten during the mission (as the astronauts are exposed to radiation), the telomeres of all three astronauts lengthened irrespective of the time spent in space.
After the astronauts returned to Earth, their telomere lengths rapidly shortened at a statistically significant rate, and a shorter average telomere length (compared to before departure) was detected. Although inter-individual differences in telomere length distributions were found, with one astronaut having a decreased number of short telomeres and an increased number of long telomeres, telomere length distributions of the 3 astronauts reveal a shift to increased number of shorter telomeres after space flight. .
Researchers speculate that space radiation triggers alternative lengthening of telomere (ALT) mechanisms. Persistent DNA Damage Response is thought to activate ALT. ALT elongates telomeres by homologous recombination, independent of telomerase. During ALT, telomerase is exported from the nucleus to the mitochondria, temporarily improving mitochondrial function, which is evidenced by increased levels of mtDNA during space flight. After space flight, when space environment-induced responses are reduced, the enzymic component of telomerase could possibly mediate the shortening of excessively long telomeres, as telomerase travels back to the nucleus from its seclusion in the mitochondria.
The study of telomeres may one day allow humans to push against the constraints of time. In this case, however, the study of telomere attrition cautions against expanding our spatial boundaries. Further studies may be able to understand and resolve health issues caused by telomere attrition, and reduce the negative effects of space travel.
References
Epel, E. S., Blackburn, E. H., & Lin, J. (2004). Accelerated telomere shortening in response to life stress. PNAS, 101(49), 17312-17315. https://doi.org/10.1073/pnas.0407162101
Garett-Bakelman, F. E., Darshi, M., & Green, S.J. (2019). The NASA twins study: A multidimensional analysis of a year-long human spaceflight. Science, 364(6436), 127. https://doi.org/10.1126/science.aau8650
Greider, C.W., & Blackburn, E. H. (1985). Identification of a specific telomere terminal transferase activity in Tetrahymena extracts. Cell, 43(2), 405-413. http://doi.org/10.1016/0092-8674(85)90170-9
Heidinger, B. J., Blount, J. D., & Boner, W. (2012). Telomere length in early life predicts lifespan. PNAS, 109(5), 1743-1748.https://doi.org/10.1073/pnas.1113306109
Luxton, J. J., McKenna, M. J., & Taylor L. E. (2020). Temporal telomere and DNA damage responses in the space radiation environment. Cell Reports, 33(10), 108435. https://doi.org/10.1016/j.celrep.2020.108435
Maestroni, L., Matmati, S., & Coulon, S. (2017). Solving the telomere replication problem. Genes, 8(2), 55. https://doi.org/10.3390/genes8020055
NASA. (2011). [The International Space Station and the Docked Space Shuttle Endeavour]. Retrieved March 1, 2022, from https://www.nasa.
Perez, J. (2017). Why space radiation matters. NASA Analogs. Retrieved October 18, 2021, from http://www.nasa.gov/analogs/nsrl/why-space-radiation-matters
Von Zglinicki, T. (2000). Role of oxidative stress in telomere length regulation and replicative senescence. Annals of the New York Academy of Sciences, 908(1), 99-110. https://doi.org/10.1111/j.1749-6632.2000.tb06639.x
Wynford-Thomas, D. & Kipling, D. (1997). The end-replication problem. Nature, 389(6651), 551. https://doi.org/10.1038/39210