In the chemical sciences, there is an enduring fascination with the art of manipulating matter. For decades, chemists have harnessed their knowledge of molecular structures to craft complex synthetic products from simple source materials, revolutionizing medicine, expanding industry, and advancing science as a whole. Yet, despite its immense potential, our current ability to make precise alterations to the connectivity of the molecular framework itself is still fairly restricted. This limitation poses considerable challenges in drug development, personalized medicine, and polymer chemistry, as it restricts access to a profusion of unexplored chemical diversity. However, numerous visionary chemists have embarked on a mission to improve the way new molecules are designed with a groundbreaking approach known as skeletal editing.
Skeletal editing is a culmination of scientific aspirations, drawing inspiration from pharmaceutical wish lists and the revolutionary genome-editing technology, CRISPR.1 This new technique enables the insertion, deletion, and substitution of individual atoms within molecules, fundamentally altering their core structures with unprecedented precision.1 It harnesses a range of tools, including modern chemical reagents, cutting-edge catalysts, and light at tuned wavelengths, to perform these molecular transformations.1 The ultimate goal is to produce a complete skeletal editing toolbox that encompasses thousands of different generalizable reactions, transcending the limitations of traditional synthesis by making atomic-level manipulation of matter an attainable reality.
Redefining Drug Discovery
Organic synthesis remains the most time-intensive step in most drug discovery projects. The primary method for identifying drug candidates for synthesis is high-throughput screening. This is a process used to assess the effects of thousands or even millions of chemical compounds on specific biological targets like receptors or enzymes.2 By screening a diverse library of compounds, researchers can identify potential drug candidates that interact with the target in a desired way, either inhibiting or enhancing its activity. However, conventional screening libraries are sourced from a limited pool of reactions and readily available materials, which restricts the spectrum of biological targets that can currently be explored. The minimal options for inspection may lead to an oversaturation of certain molecular structures within pharmaceuticals.3 This issue is largely attributed to contemporary synthesis strategies emphasizing peripheral editing techniques. Skeletal editing offers a solution by facilitating the functionalization and refinement of underlying molecular skeletons in the later stages of synthesis, offering more convenient access to valuable synthetic transformations that were previously considered impractical or unattainable.
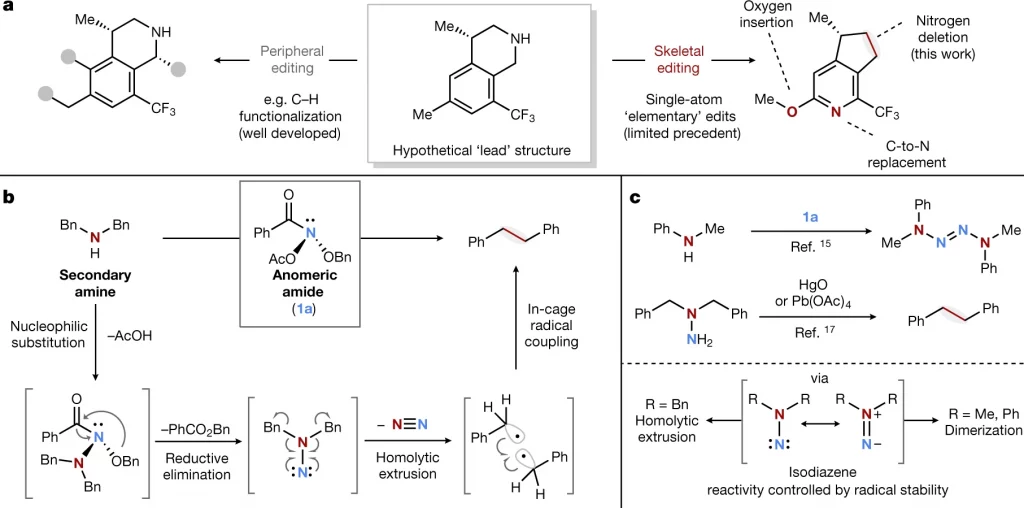
Once a drug candidate and its corresponding synthesis pathway have been identified, medicinal chemists embark on synthesizing analogs, which are chemical compounds that are structurally similar to an original compound, but contain slight differences in functional groups or other components. Each analog is aimed at improving crucial drug characteristics, such as binding affinity, selectivity, or potency.4 This stage requires the chemists to map out a series of reactions producing the molecular changes that would lead to the desired products. However, translating these conceptual changes into reality often presents its own challenge. Creating new syntheses for each of these analogs from the ground up is both time-consuming and costly. Skeletal editing provides an ingenious alternative. In a more pragmatic approach to deriving analogs, skeletal editing has the capacity to add or remove specific atoms or functional groups, which are specific combinations of atoms within molecules that determine their characteristic chemical reactivity, at the end of a synthesis. This means there is no longer the need for complete resynthesis each time.
The vast majority of drug molecules today are characterized by the presence of numerous closely positioned polar functional groups, meaning they have unevenly distributed electron density that results in partial charges. 5 These strategically placed polar elements are intentionally incorporated in bioactive molecules to facilitate strong binding interactions with target enzymes or proteins, producing desirable drug-like properties.5 However, the synthesis of these drug candidates and their desired analogs present significant challenges to modern chemists.
Achieving precise control over the formation and manipulation of these specific chemical groups demands sophisticated synthetic methodologies and careful design considerations. This is to ensure the desired molecular outcomes while avoiding unwanted side reactions or byproducts stemming from common polar constituents’ inherent reactivity and complex structural nature. The skeletal editing approach circumvents the issues associated with reactions between polar groups and the catalysts and chemical reagents commonly used in synthesis. In application, skeletal editing develops reactions that enable the introduction of specific functional groups into a molecule during the late stages of synthesis, after the primary carbon backbone has already been constructed. These new reactions eliminate the need to initiate an entirely new synthetic route for each target molecule by enabling unconventional disconnections and the selective addition or deletion of small chemical groups at each position of the lead molecule of interest.
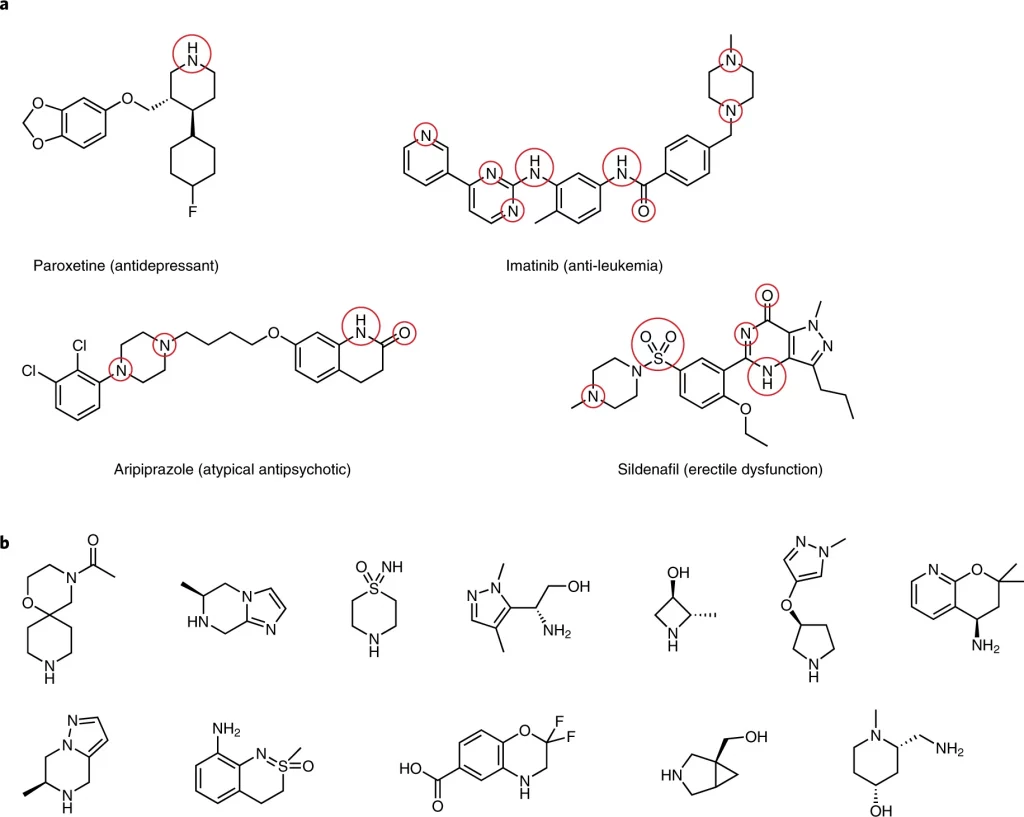
An exciting application of this methodology is in the modification of natural products, which are chemical compounds derived from living organisms, often possessing biological activity. Importantly, natural products have an extensive and proven track record as therapeutic agents. Research suggests that nearly 50% of all anti-cancer medications introduced between the 1940s and 2014 were either derived from or influenced by natural products.5 Natural products are unique in their ability to explore the biologically relevant chemical space, making them valuable leads for drug candidates. Unfortunately, there is a reduced interest in these molecules from pharmaceutical companies due to the technical challenges associated with the high-throughput screening and synthesis of natural products. The most advanced techniques for the synthesis of natural products are transition-metal catalyzed cross-coupling reactions, carbon–hydrogen bond functionalization reactions, and selective ring formation.6 These techniques are known for their complexity and typically require multiple steps to create compounds with drug-like functionalities. In response, the concept of skeletal editing, involving the direct insertion, deletion, or exchange of atoms, presents a valuable opportunity to selectively manipulate intricate natural products. This capability would greatly expand the versatility of screening libraries, enabling them to yield hits for more challenging screening targets, such as protein-protein interactions. It would also expedite the production of analogs of complex scaffolds, such as those found in natural products.7
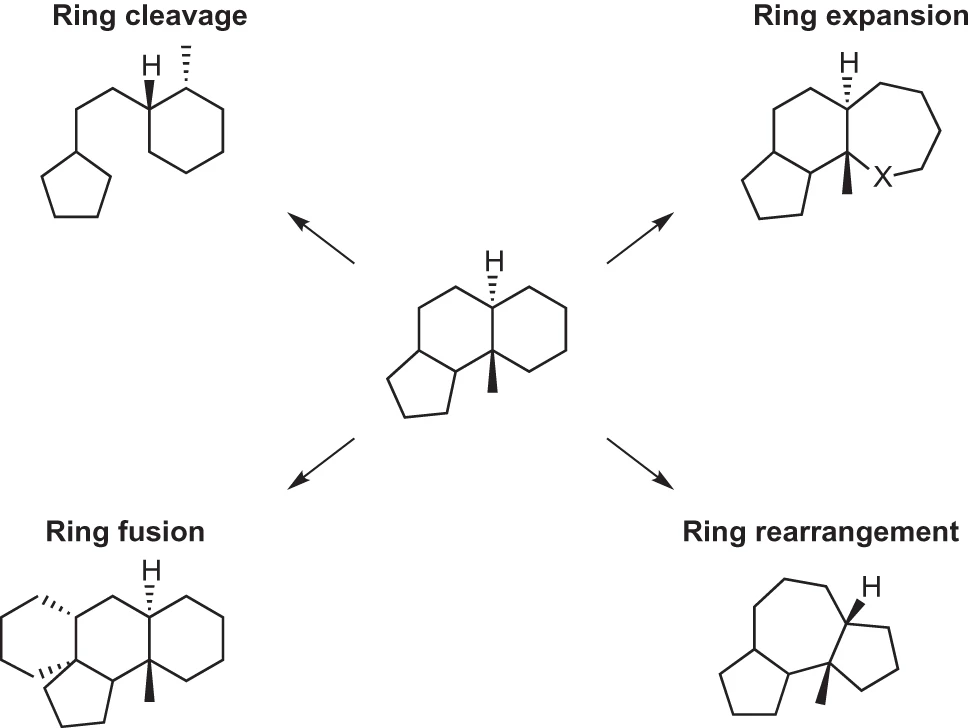
Custom Cures on the Horizon
Imagine a world where cancer treatment isn’t a one-size-fits-all approach, but a tailored therapy designed specifically for the individual. Advancing personalized medicine, skeletal editing holds the promise of facilitating the rapid and precise construction of therapeutic molecules. These meticulously designed compounds possess the capacity to selectively target the unique characteristics of a patient’s tumor, ensuring maximum efficiency while minimizing potential side effects.8 This innovative approach to molecular design has the potential to fine-tune drug molecules to meet the specific needs of individual patients. It enables the creation of precise binding mechanisms and the optimization of bioavailability, paving the way for personalized medicine.
Sculpting the Future
The rise of skeletal editing research signifies a profound paradigm shift in the fields of organic and synthetic chemistry. Its diverse range of molecular editing techniques—spanning ring expansion, contraction, and single-atom insertion and deletion—offers a level of precision and control over molecular structures that were formerly impossible.9 Skeletal editing does, however, face some challenges with efficiency, as certain reactions transform only a fraction of the reacted molecules into the intended product. The transformative process also often uses highly reactive reagents and the preliminary installation of additional molecular fragments, shielding fragile functional groups during the reaction.1 This can lead to the generation of undesirable by-products, consequently demanding more intricate purification procedures to obtain the ultimate product. Nevertheless, with ongoing advancements in the identification of common reaction motifs, skeletal editing reactions are becoming more generalizable, expanding the scope of the skeletal editing toolbox. This technique holds the promise of a revolution in organic molecule synthesis and groundbreaking medicines, alluding to an era where automated synthesis laboratories and tailored therapies will become standard. Skeletal editing is a testament to human ingenuity, ready to reshape our world in ways we’re only beginning to understand.
Acknowledgments
I would like to express my sincere gratitude to Cassandra Sedler, a researcher with Dr. Sarah Wengryniuk’s lab at Temple University, for their invaluable feedback and contributions to enhancing the quality of this article.
References
- Peplow, M. (2023). “Almost magical”: chemists can now move single atoms in and out of a molecule’s core. Nature, 618(7963), 21–24. https://doi.org/10.1038/d41586-023-01735-1
- Huigens, R. W., Morrison, K. E., Hicklin, R. W., Flood, T. A., Richter, M. F., & Hergenrother, P. J. (2013). A ring-distortion strategy to construct stereochemically complex and structurally diverse compounds from natural products. Nature, 5(3), 195–202. https://doi.org/10.1038/nchem.1549
- Boström, J., Brown, D. G., Young, R. J., & Keserü, G. M. (2018). Expanding the medicinal chemistry synthetic toolbox. Nature Reviews Drug Discovery, 17(10), 709–727. https://doi.org/10.1038/nrd.2018.116
- Brown, D. G., & Boström, J. (2015). Analysis of Past and Present Synthetic Methodologies on Medicinal Chemistry: Where Have All the New Reactions Gone? Journal of Medicinal Chemistry, 59(10), 4443–4458. https://doi.org/10.1021/acs.jmedchem.5b01409
- Blakemore, D. C., Castro, L., Churcher, I., Rees, D. C., Thomas, A. W., Wilson, D. M., & Wood, A. (2018). Organic synthesis provides opportunities to transform drug discovery. Nature Chemistry, 10(4), 383–394. https://doi.org/10.1038/s41557-018-0021-z
- Hui, C., Wang, Z., Wang, S., & Xu, C. (2022). Molecular editing in natural product synthesis. Organic Chemistry Frontiers, 9(5), 1451–1457. https://doi.org/10.1039/D2QO00043A
- Harvey, A. L., Edrada-Ebel, R., & Quinn, R. J. (2015). The re-emergence of natural products for drug discovery in the genomics era. Nature Reviews Drug Discovery, 14(2), 111–129. https://doi.org/10.1038/nrd4510
- Miles, N. C. (2023, August 5). “Endless possibilities”: the chemists changing molecules atom by atom. The Observer.https://www.theguardian.com/science/2023/aug/05/skeletal-editin g -chemistry-molecule-atom-medicine-drug-development-sustainable
- Kennedy, S. H., Dherange, B. D., Berger, K. J., & Levin, M. D. (2021). Skeletal editing through direct nitrogen deletion of secondary amines. Nature, 593(7858), 223–227. https://doi.org/10.1038/s41586-021-03448-9
Image References
Banner Image: Molecules and biology, biological concept, 3d rendering. Computer… (2020, March 23). IStock. https://www.istockphoto.com/photo/molecules-and-biology-biological-concept-3d-rendering-gm1213068546-352386851?phrase=medicinal+chemistry
Figure 1: Kennedy, S. H., Dherange, B. D., Berger, K. J., & Levin, M. D. (2021). Skeletal editing through direct nitrogen deletion of secondary amines. Nature, 593(7858), 223–227. https://doi.org/10.1038/s41586-021-03448-9
Figure 2: Blakemore, D. C., Castro, L., Churcher, I., Rees, D. C., Thomas, A. W., Wilson, D. M., & Wood, A. (2018). Organic synthesis provides opportunities to transform drug discovery. Nature Chemistry, 10(4), 383–394. https://doi.org/10.1038/s41557-018-0021-z
Figure 3: Huigens, R. W., Morrison, K. E., Hicklin, R. W., Flood, T. A., Richter, M. F., & Hergenrother, P. J. (2013). A ring-distortion strategy to construct stereochemically complex and structurally diverse compounds from natural products. Nature, 5(3), 195–202. https://doi.org/10.1038/nchem.1549