INTRODUCTION
Breast cancer is the world’s most common cancer, with over 300,000 new cases diagnosed yearly in the United States alone. For localized, or non-metastasized, tumor removal surgery is the most widespread treatment method for breast cancer. However, due to non-optimal cancer visualization techniques during surgery, the re-excision rates for breast cancer (the need for a second surgery) are around 20-30%.1 Re-excision is not only invasive and costly, but increases patient anxiety, affects cosmetic results, and opens doors for wound infection.2 That being said, it is imperative for the healthcare industry to make strides in reducing these overall re-excision rates. This goal is materializing through the use of near infrared technology, which allows for more concrete cancer visualization.
THE UNMET NEED
During the early stages of breast cancer, cancerous cells conglomerate into a tumor within breast tissues. While performing breast cancer surgery, surgeons map out a resection margin that demarcates the tumor from healthy tissue.3 Surgeons then cut the tumor out using the resection margin as a guide. In order to create the most accurate resection margin, surgeons adhere to minute visual cues to identify differences between cancerous and healthy regions. These differences could be chemical or physiological and include variations in color, bleeding patterns, cell density, and calcium deposition.2
A major problem, however, is that surgeons are often left to their professional judgment and the naked eye to find the tumor’s edges. If the tumor margin is especially ambiguous, then surgeons may accidentally leave cancerous cells and cell conglomerates behind. As Dr. Daniel Orringer, a surgeon at the University of Michigan, claims, “in a lot of cases, we leave tumors behind that could be safely removed if only we were able to better visualize it.” 4 Lingering cancer cells can continue to divide and risk the formation of new tumors post-operation, contributing to high re-excision rates.
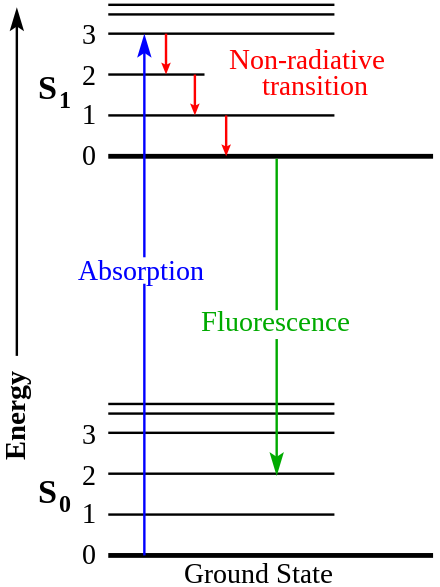
New and effective ways of visualizing cancerous cells during surgery can prevent cancerous cells from lingering post-op and decrease re-excision rates. Through the use of near infrared (NIR) light beams, surgeons have the ability to view cancerous cells in real-time during operation and remove them accordingly, thus eliminating the need for external pathology reviews or underestimating resection margins. One of these specific NIR visualization tools involves the creation of lab-made crystalline molecules known as rare-earth nanoprobes.
HOW THE PROBES EMIT LIGHT
Rare-earth nanoprobes are tiny, lab-made vessels composed primarily of rare earth elements, such as ytterbium, erbium, holmium, thulium, and praseodymium. These atoms are collectively known as lanthanides, and possess important visual properties.5 When exposed to a specific form of near-infrared light (NIR), with a wavelength of 980 nm, rare earth elements fluoresce bright colors. How does this fluorescence work? In the atomic model, negatively charged electrons are constantly moving around the atom’s positively charged nucleus in orbitals of varying densities and locations. When exposed to an energy source like NIR light, the electrons of rare earth elements gain energy and jump to a higher energy orbital, thus overcoming nuclear attractive forces. The process of energy absorption is known as electron excitation. However, given that lower energy states are more thermodynamically stable, electrons eventually fall back to a lower energy sublevel, releasing energy as they return to their original state.6 While some of this emitted energy is dissipated within the environment, the rest of it is released in the form of short-wave infrared light waves (SWIR). Due to SWIR’s high reflectivity and penetrability, humans can easily perceive SWIR emanating from the probes in the form of brightly colored fluorescence while wearing infrared goggles.
BIOCOMPATIBILITY AND CANCER DETECTION
Typically, rare earth nanoprobes are rejected by the human body because they cannot be naturally metabolized. However, albumin, a liver protein that assists in moving small molecules through blood, has the ability to make these nanocrystals biocompatible. Once encompassed within an albumin membrane, the human body can easily intake and transport these probes without flagging them as foreign molecules.
Proteins like albumin are composed of amino acids which possess specific chemical groups, called functional groups. The functional groups are composed of different atoms and charges and have the ability to bind to other molecules, such as a cancer antibody. Antibodies are small proteins that attach to receptors on threat-posing substances—in this case, harmful cancer cells. Many cancer antibodies are known by scientists and can be commercially purchased or produced in a lab. Through capitalizing on the bonding affinity between functional groups, the albumin-bound rare earth nanoprobes can be bonded to these antibodies before injection into the body. These nanoprobe-antibody conjugates will then be transported throughout the human body and bond to only cancerous cells where they can fluoresce under NIR exposure.
POTENTIAL IMPLICATIONS
Once an incision is made in the breast during breast cancer surgery, surgeons can inject nanoprobes directly into the tumor so that they can bond to cancer cells and pre-metastatic lesions along the resection margin within a few minutes. After shining a NIR beam over the surgical site, surgeons will be able to identify all of the fluorescing cancer cells under a screening device and easily remove them, thus greatly reducing the need for re-excision. Following surgery, nanoprobes will leave the body naturally within two days in the form of excretion.
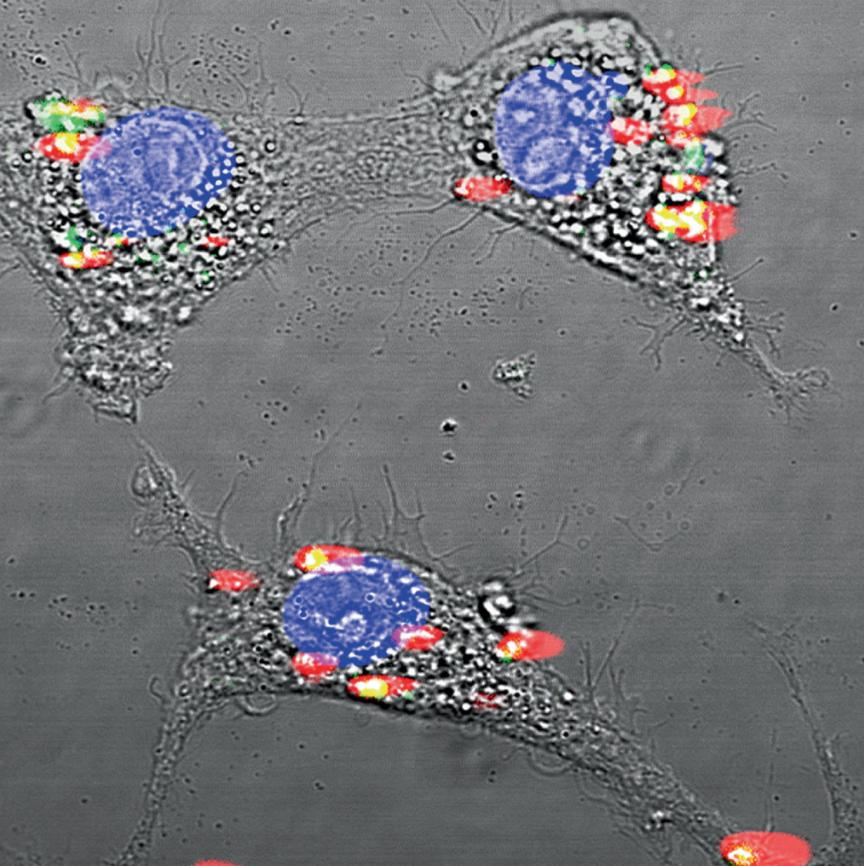
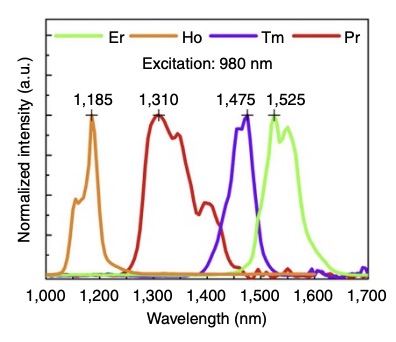
FUTURE USES
Within smaller mammals, such as mice, rare-earth nanoprobes can also be used as a general pre-emptive cancer detection device. Rather than resorting to invasive biopsies or expensive MRI scans, the nanoprobes can be injected intravenously into the mouse and travel around the body while attaching to undetected cancer cells. Given that each rare earth element emits a different wavelength of SWIR, and thus a slightly different color after exposure to NIR, these nanoprobes can be “programmed” to glow a distinct color when attached to a specific cancer, whether breast cancer, melanoma, or leukemia. This means that preemptive cancer detection could potentially happen in one sweep. However, since humans are much larger in size and more anatomically complex than mice, further research is needed to see if rare earth nanoprobes could provide efficient and accurate means of multi-faceted cancer detection in humans.
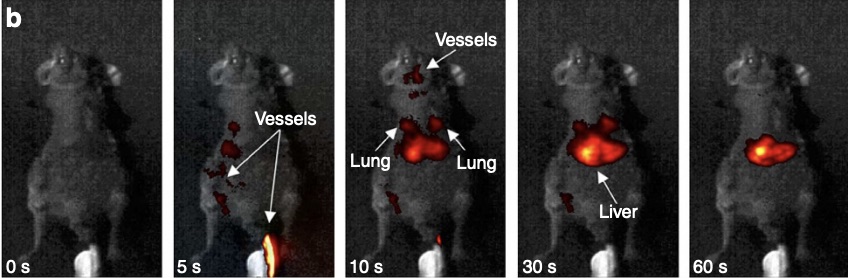
CONCLUSION
It is widely known that the most proactive way to treat cancer is to detect it early. In terms of breast cancer, the five year-relative survival rate for Stages 0-1 is almost 100%, whereas the survival rate for Stage 4 is less than 28%.7 To prevent late-stage progression, it is imperative that cancer cells are thoroughly removed during the first surgery and are not left to linger and divide further. Through the use of near infrared technology, visualization devices like rare earth nanoprobes have the ability to assist in the complete removal of cancer cells, thus increasing relative survival rates and revolutionizing the field of cancer altogether.
ACKNOWLEDGMENTS
I would like to thank Tong Zhan, a chemical biology Ph.D. candidate at the University of California, Berkeley, for reviewing this article and offering insightful feedback.
REFERENCES
- Elmore L.C., Margenthaler J.A. (2019, December). A tale of two operations: re-excision as a quality measure. Gland Surgery. Retrieved October 9, 2023, from https://gs.amegroups.org/article/view/32941/27655
- Jung W., Kang E., Kim S.M., Kim D., Hwang Y., Sun Y., Yom C.K., Kim S.W. (2012, December). Factors ASsociated with Re-excision after Breast-Conserving Surgery for Early-Stage Breast Cancer. Journal of Breast Cancer. Retrieved October 9, 2023, from https://ejbc.kr/DOIx.php?id=10.4048/jbc.2012.15.4.412
- Orosco R.K., et al. (2018, April 9). Positive Surgical Margins in the 10 Most Common Solid Cancers. Scientific Reports. Retrieved October 9, 2023, from https://www.ncbi.nlm.nih.gov/pmc/articles/PMC5890246/#
- National Institute of Health. (2016). Technologies Enhance Tumor Surgery: Helping Surgeons Spot and Remove Cancer. News in Health. Retrieved October 9, 2023, from https://newsinhealth.nih.gov/2016/02/technologies-enhance-tumor-surgery
- Naczynski D.J., Tan M.C., Zevon M., Wall B., Kohl J., Kulesa A., Chen S., Roth C.M., Riman R.E. & Moghe P.V. (2013, July 22). Rare-earth-doped biological composites as in vivo shortwave infrared reporters. Nature Communications. Retrieved October 9, 2023, from https://www.nature.com/articles/ncomms3199.pdf
- Iowa State University. (n.d.). Why Things Fluoresce. Center for Nondestructive Evaluation. Retrieved October 9, 2023, from https://www.nde-ed.org/NDETechniques/PenetrantTest/PTMaterials/whythingsfluoresce.xhtml
- Kandola, Aaron. (2023, March 3). What are the most curable cancers? Medical News Today. Retrieved October 9, 2023, from https://www.medicalnewstoday.com/articles/322700#:~:text=1.,as%20people%20without%20the%20condition.
IMAGE REFERENCES
Header Image: Grayson, K. (2019, November). Glass 101: Making Fluorescent Glass with Rare Earth Oxides. Mo-Sci Corporation. Retrieved October 9, 2023, from https://mo-sci.com/fluorescent-glass-rare-earth-oxides/
Figure 1: Fluorescence. (2023, November 8). In Wikipedia. https://en.wikipedia.org/wiki/Fluorescence
Figure 2: Naczynski D.J., Tan M.C., Zevon M., Wall B., Kohl J., Kulesa A., Chen S., Roth C.M., Riman R.E. & Moghe P.V. (2013, July 22). Rare-earth-doped biological composites as in vivo shortwave infrared reporters. Nature Communications. Retrieved October 9, 2023, from https://www.nature.com/articles/ncomms3199.pdf
Figure 3: Blesch C. (2014, October 21). Rare earth nanocrystals and infrared light can reveal small cancerous tumors and cardiovascular lesions. Rutgers Today. Retrieved October 9, 2023, from https://www.rutgers.edu/news/detecting-cancer-earlier-goal-rutgers-developed-medical-imaging-technology