You are running around a track—legs in stride, arms pumping, chest rising and falling in rhythm. From the perspective of someone watching from the bleachers, it is evident that there are external effects of this exertion: sweat drips from your brow, blood rushes to your cheeks, and your breath comes quick and sharp.
However, what is less clear is the flurry of molecular activity that is happening internally. Signaling pathways increasing the production or changing the activity of muscle proteins are triggered, cascades of microscopic-sized hormones are secreted, rates of glycogen breakdown and ATP synthesis are intensified, and inflammatory and immune marker expressions are modulated.
In short, your body is reshaping itself: its organs, tissues, and cellular pathways are transformed in response to physical exercise.
We Know Exercise Is Good for Our Bodies… What Is New?
The scientific community has accumulated substantial data showing that regular exercise results in various health benefits for humans. These include everything from preventing cardiovascular and neurological diseases, to reducing risk of cancer, to alleviating anxiety or depression.1,3
However, as much as the body’s positive responses to exercise have been documented, what has largely eluded scientists is how these physiological effects arise; this requires digging deeper and examining the body at the molecular level. Understanding these molecular mechanisms underlying how organs benefit in response to exercise is vital to developing medications that can improve human health and mimic the positive effects of exercise for individuals with physical activity limitations. Moreover, gaining molecular insights into how exercise helps prevent specific diseases could aid in producing targeted therapies for these diseases. Yet, studies in this field have been generally narrow in scope and limited in depth—at least, until earlier this year.
New Research Plunges Us into the Molecular World of Exercise
In 2016, the Molecular Transducers of Physical Activity Consortium (MoTrPAC) was established by a group of scientists from leading research institutes nationwide, including Stanford University and the Broad Institute of MIT and Harvard. The team aims to develop a molecular “map” of the body’s integrated response to exercise training through interdisciplinary data collection and analytics. In May 2024, the consortium published their most recent findings in a series of articles in Nature.
To investigate the molecular mechanisms of exercise response, the study developed a robust system of progressive treadmill endurance (aerobic) exercise training for six-month-old male and female rats. The rats were placed on treadmills five days a week for one, two, four, or eight weeks and subjected to increasing exercise intensity.1,2,4 Samples from 18 different tissues, blood, and plasma were then collected from the rats 48 hours after their latest bout of exercise and distributed to the consortium’s labs across the country, where they were put through an extensive range of experiments and tests.2 Thus, researchers could comprehensively study the rats’ adaptive molecular responses to endurance training rather than their acute ones. In essence, the study focused on how the animals’ bodies changed and responded to the stress of physical exertion over days rather than immediately after training.
To analyze their samples, the MoTrPAC team integrated various “omic” platforms that recorded the comprehensive biomolecular processes taking place in response to exercise. Namely, the group gathered extensive and eclectic molecular data by measuring changes in the transcriptome (full range of RNA molecules being transcribed from DNA), proteome (array of proteins present in a cell), metabolome (complete set of metabolites), and immunome (genes and proteins in the immune system), among other “omes,” in the rats’ tissue samples.2
Prior studies in this field over the past few decades have examined the molecular mechanisms behind exercise’s benefits in the human model, instead of in rats—extensive research, for instance, has been done on humans’ skeletal muscular adaptations to exercise, including significant epigenetic changes in DNA methylation and gene expression.
However, these past studies mainly focused on only a single tissue type utilizing generally one or two omic platforms; in contrast, MoTrPAC’s work presented the first whole-organism, multi-tissue, and multi-omic molecular map of the temporal effects of exercise. Researchers performed over 10,000 assays and 15 million measurements on blood and 18 tissues (including the heart, skeletal muscle, liver, kidney, brain, lung, and brown and white adipose, or fat, tissues) across 294 rats, identifying thousands of molecules and cellular pathways altered by exercise.4,5 These alterations depended on both the number of weeks the rats had been exercising and their sex.
Although MoTrPAC is currently conducting human studies, the molecular map developed in rats is an ideal model for our responses to exercise. Rats and humans share remarkable physiological, metabolic, and anatomical similarities in their organ structure and function; furthermore, genomic sequencing reveals that rats have about the same number of genes as humans, with almost all human genes linked to diseases having highly conserved counterparts in the rat genome.4,6,7 Nonetheless, researchers cannot map exercise’s molecular effects in rats directly to humans, and thus, the study does not allow for any absolute conclusions about the human body.
However, this was not its aim; MoTrPAC strived to build a platform that fuels future hypothesis-driven research. Following their Nature articles, the team established a free, online public database and tissue biorepository of all the molecular alterations they measured in the rats—a comprehensive tool that can be used by other researchers to identify promising molecular targets to further explore in humans.
The Body, Transformed
Several key findings in MoTrPAC’s study illuminate exciting research targets and provide glimpses into just how complex the body’s molecular adaptations to exercise could be.
The MoTrPAC team identified a total of 7,115 unique genes across the rat tissues that exhibited specific exercise-responsive changes in their regulation, with 67% of these genes being tissue-specific.1 This means that not only did the expression of thousands of different genes change after the rats exercised, but most of these genes were selectively expressed in only certain cell types.
The genes led to a myriad of changes in pathways unique to different cell types, including tissue remodeling in the lungs, alterations in ion flux in the heart and organelle biogenesis (synthesis), and maintenance in the blood.1 Many exercise-responsive genes were also shared between different tissues, including 249 genes in lung and white adipose tissue that caused enrichments in pathways ranging from immune cell recruitment to decreased inflammatory responses.1 Significantly, 22 exercise-regulated genes that, among other things, induced heat shock proteins (HSPs)—which maintain cell metabolic function and protect cells from stress—were identified across white adipose, liver, skeletal muscular, heart, lung, and kidney tissues.1,8
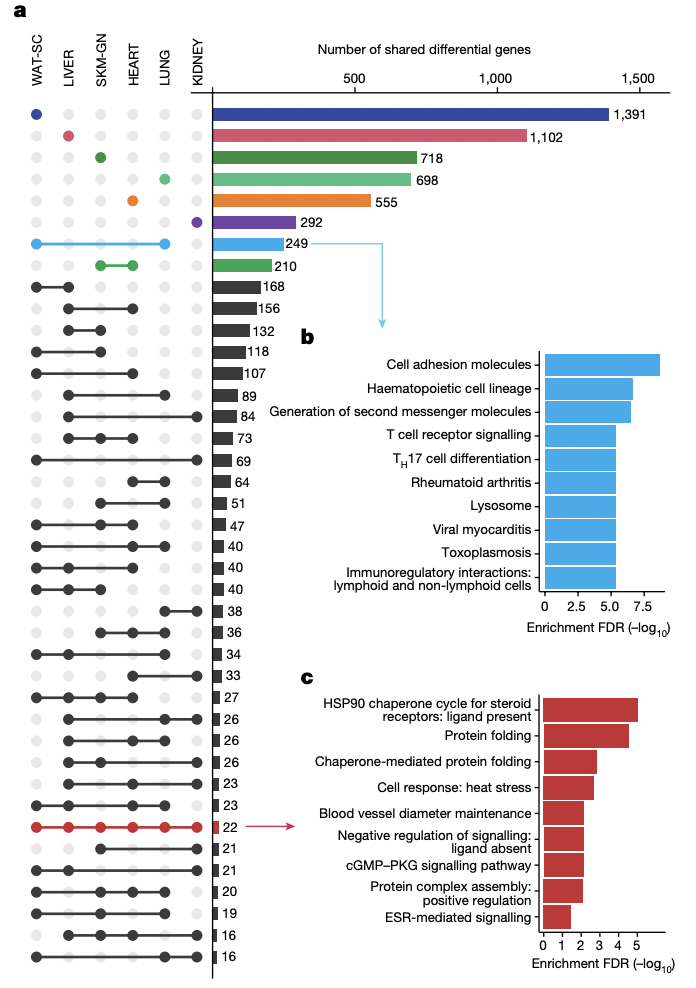
a) Plot depicting number of exercise-regulated genes across six different rat tissues. Singular dots indicate tissue-specific genes, while bars connecting dots represent exercise-regulated genes shared between 2 or more tissues.
b) Different cellular pathway enrichments caused by the 249 exercise-regulated genes (shown in blue) shared between subcutaneous white adipose (WAT-SC) and lung (LUNG) tissues.
c) Different cellular pathway enrichments (shown in red) caused by the 22 exercise-regulated genes shared between all six tissues considered in (a).
The MoTrPAC team also identified changes in the abundance of numerous transcription factors across many rat tissues after exercise. This included a group involved in vascular development and neuronal differentiation in the heart and skeletal muscle.1 Additionally, the team observed increased phosphorylation of key regulators involved in cell proliferation in the liver, suggesting potential mechanisms behind liver regeneration in response to exercise.1
While these and countless other molecular changes recorded in MoTrPAC’s study further our understanding of the body’s response to exercise, certain discoveries were of particular interest to the group, as they could provide exciting insights into how exercise helps prevent or manage prevalent human diseases.
In the small intestine of the rats studied, MoTrPAC researchers observed decreased expression of transcripts with genetic risk loci for inflammatory bowel disease (IBD), a group of autoimmune diseases that result from the immune system attacking its own digestive system.1 These down-regulated transcripts were from genes such as Cxcr3 and Il1a, which encode proteins involved in immune response, inflammation, and the development of IBD.1 Such observations yield critical insights into exercise’s role in decreasing the risk of IBD and provide potential molecular targets to address it.
MoTrPAC researchers were also highly interested in findings from the liver. Leveraging metabolomic analysis techniques, the team found that exercise increased protein abundance and acetylation in the liver’s mitochondrial, amino acid, and lipid metabolic pathways.1 Additionally, the team saw increases in a phosphorylation signal that regulates liver energy storage and the acetylation of proteins related to the peroxisome, an organelle heavily involved in lipid metabolism.1,5 These extensive molecular adaptations in the liver could help explain how exercise improves liver health and prevents fat accumulation in the organ.1,5 This could have high-potential implications for researchers studying non-alcoholic fatty liver disease (NAFLD), the most common chronic liver disorder in the U.S. that affects about 25% of U.S. adults.9,10
Moving into the Future
Despite the incredible advances achieved by the MoTrPAC study, much work remains.
The team is currently conducting studies that examine the acute effects of physical activity on the same rat organs and tissues they focused on in the recent study.2 Furthermore, MoTrPAC has begun human studies to investigate the multi-tissue, temporal, and sex-specific effects of endurance and resistance training in adults and children; these studies could point more directly to molecular targets for exercise mimetic drugs and treatments for human diseases.5
Nevertheless, the recent study has provided an invaluable public database for future hypothesis-driven investigations to launch. It has gleaned novel insights into the molecular networks behind exercise’s physical benefits on a scale not previously witnessed. It could help reveal how a seemingly simple and mundane activity, like running around a track, has transformative internal effects on our bodies.
And we are just at the start of the race.
Acknowledgments
I would like to thank Carl Johan Sundberg, MD, PhD, who is a Professor in the Department of Physiology and Pharmacology and leads the Molecular Exercise Physiology research group at the Karolinska Institutet in Solna, Sweden, for generously taking the time to review my article for accuracy and providing me with valuable feedback.
References
- MoTrPAC Study Group., Lead Analysts. & MoTrPAC Study Group. Temporal dynamics of the multi-omic response to endurance exercise training. Nature 629, 174–183 (2024). https://doi.org/10.1038/s41586-023-06877-w
- Endurance exercise causes a multi-organ full-body molecular reaction. (2024). Nature. https://doi.org/10.1038/d41586-024-00585-9
- Mayo Foundation for Medical Education and Research. (2023, August 26). 7 great reasons why exercise matters. Mayo Clinic. https://www.mayoclinic.org/healthy-lifestyle/fitness/in-depth/exercise/art-20048389
- Simon Schenk, Tyler J Sagendorf, Gina M Many, Ana K Lira, Luis G O de Sousa, Dam Bae, Michael Cicha, Kyle S Kramer, Michael Muehlbauer, Andrea L Hevener, R Scott Rector, John P Thyfault, John P Williams, Laurie J Goodyear, Karyn A Esser, Christopher B Newgard, Sue C Bodine, The MoTrPAC Study Group , Physiological Adaptations to Progressive Endurance Exercise Training in Adult and Aged Rats: Insights from the Molecular Transducers of Physical Activity Consortium (MoTrPAC), Function, Volume 5, Issue 4, 2024, zqae014, https://doi.org/10.1093/function/zqae014 OR Physiological Adaptations to Progressive Endurance Exercise Training in Adult and Aged Rats: Insights from the Molecular Transducers of Physical Activity Consortium (MoTrPAC). (2024). Function, 5(4).
- DiCorato, A. (2024a, May 1). Scientists work out the effects of exercise at the cellular level. Broad Institute. https://www.broadinstitute.org/news/scientists-work-out-effects-exercise-cellular-level
- National Human Genome Research Institute. (2004). Scientists compare rat genome with human, mouse. Genome.gov. https://www.genome.gov/11511308/2004-release-scientists-compare-rat-genome#:~:text=In%20their%20Nature%20paper%2C%20the,patterns%20across%20the%20organisms’%20chromosomes.
- Bryda, E. (2013). The Mighty Mouse: The Impact of Rodents on Advances in Biomedical Research. Missouri Medicine – The Journal of the Missouri State Medical Association, 110(3).
- ScienceDirect. Heat Shock Protein. Heat Shock Protein – an overview | ScienceDirect Topics. https://www.sciencedirect.com/topics/biochemistry-genetics-and-molecular-biology/heat-shock-protein
- U.S. Department of Health and Human Services. (n.d.). Definition & Facts of NAFLD & NASH. National Institute of Diabetes and Digestive and Kidney Diseases. https://www.niddk.nih.gov/health-information/liver-disease/nafld-nash/definition-facts
- A Silent Epidemic: 25% of Americans Have Fatty Liver Disease | Lee Health. Lee Health. (2023). https://www.leehealth.org/health-and-wellness/healthy-news-blog/health-hub/non-alcoholic-fatty-liver-disease-25-of-americans
Image References
- Banner Image: Job-Reese, R. (2024). Broad Institute. Broad Communications. Retrieved from https://www.broadinstitute.org/news/scientists-work-out-effects-exercise-cellular-level.
- Figure 1: MoTrPAC Study Group., Lead Analysts. & MoTrPAC Study Group. Temporal dynamics of the multi-omic response to endurance exercise training. Nature 629, 174–183 (2024). https://doi.org/10.1038/s41586-023-06877-w
- Figure 2: MoTrPAC Study Group., Lead Analysts. & MoTrPAC Study Group. Temporal dynamics of the multi-omic response to endurance exercise training. Nature 629, 174–183 (2024). https://doi.org/10.1038/s41586-023-06877-w
- Figure 3: MoTrPAC Study Group., Lead Analysts. & MoTrPAC Study Group. Temporal dynamics of the multi-omic response to endurance exercise training. Nature 629, 174–183 (2024). https://doi.org/10.1038/s41586-023-06877-w