A Bite of Ice Cream
Imagine you have spent the past week locked in a library studying for midterms, sat through hours of dense lectures, and have just gotten back from a grueling day at work. To ease the tension, you reach into the freezer for a tub of ice cream. It’s mint chocolate chip, your absolute favorite. As you take a bite, you smile and the day feels a little brighter. That satisfying feeling comes from a minuscule molecule called dopamine.
While dopamine is best known as the chemical responsible for the satisfying feeling from rewards like ice cream, it is also critical for the function of many parts of the brain, from mood to motion. Many of our voluntary movements are influenced by dopamine, including opening the freezer and lifting the spoon to grab a scoop of ice cream. Though we may not be conscious of its role, the chemical pathways dopamine is involved in underlies many of our essential functions.
However, the mechanisms by which such complex networks of neurons carry out these fine motor tasks and the potential problems that may arise when they fail is still unclear to researchers. An approach to answering this question is by first understanding the development of neurons and their organization
The Birth of Neurons
The life of a neuron begins in the neuroectoderm, a portion of the ectoderm. The ectoderm is the outermost layer of tissue in embryos during early development. Through the process of neurulation, these ectoderm cells make up the neural plate. This plate folds inward to fuse into a tube structure, aptly named the neural tube. The neural tube eventually differentiates into sections of the nervous system, such as the spinal cord and midbrain. During neurulation, proteins called transcription factors promote the differentiation of midbrain floor plate (mFP) cells into midbrain dopamine (mDA) neurons. These transcription factors regulate gene expression within the cell such that only the genes that are expressed in dopamine neurons are transcribed, aiding in its specialization into a mDA neuron.4
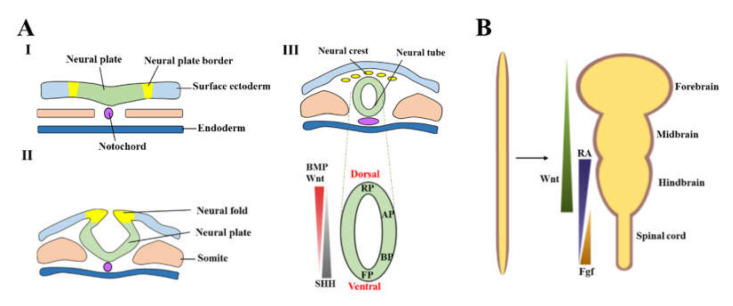
The Functional Pathway
Dopamine is essential in many pathways in the brain. Some, such as the mesolimbic pathway, correspond to our pleasure and reward function, while the nigrostriatal pathway is tied to voluntary motor function. The nigrostriatal dopamine pathway is primarily located in the medial temporal lobe, within a structure of the brain critical for movement called the basal ganglia. Like a cargo transport highway, this pathway synthesizes dopamine in the substantia nigra, a small structure in the basal ganglia. These molecules of dopamine then travel to the caudate and putamen, which make up the dorsal part of the striatum — this brain region is a cluster of nuclei within the basal ganglia.2
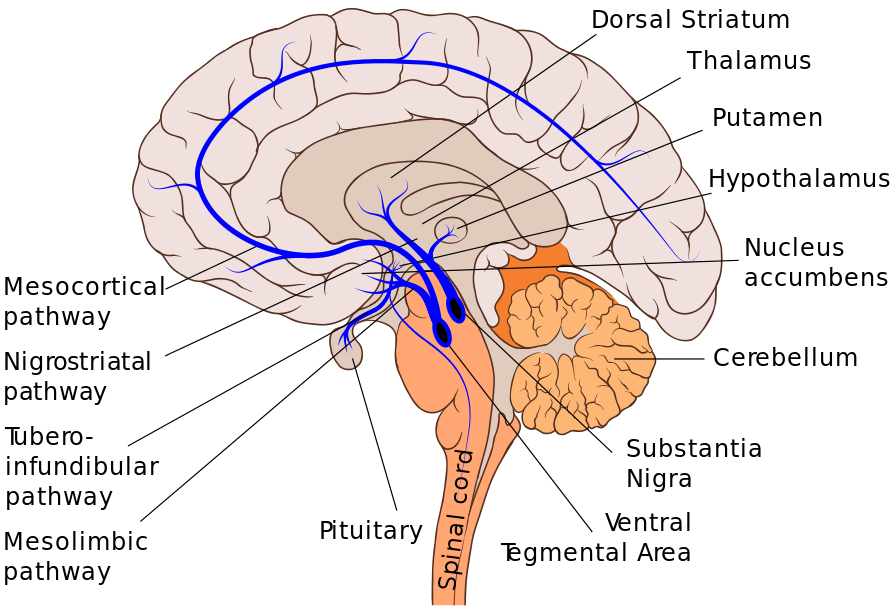
The Loss of Dopamine
When this dopamine circuit becomes weakened, people experience serious problems in movement and coordination. One such disease tied to the nigrostriatal pathway is Parkinson’s disease. This neurodegenerative disease involves the death of dopaminergic neurons (mDA) in the brain. In particular, dopamine neurons in the substantia nigra—where dopamine is produced and exported in the movement pathway—are lost. As a result, individuals suffering from Parkinson’s disease exhibit tremors and a slowness of movement. There are many hypotheses for the cause of neuronal death in these regions including oxidative stress, mutations in proteins, and lysosomal dysfunction.1 The leading hypothesis today is that apoptosis or programmed cell death of dopamine neurons is caused by toxic aggregations of a misfolded protein called alpha-synuclein (p.α-syn). These aggregates hinder mDA neurons in multiple ways, including impairing transport of signaling molecules within the cell and slowing the release of dopamine by the neuron, before eventually leading to neuronal death.2
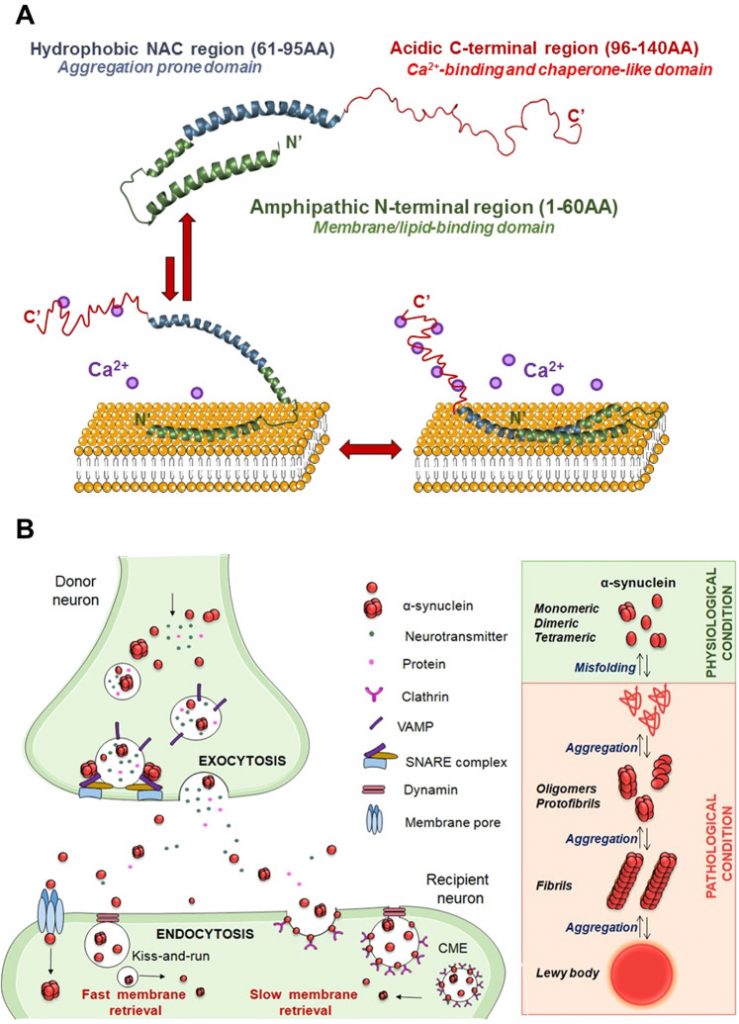
Tragically, as humans age, brain diseases such as Parkinson’s become more prevalent. Neuronal death has serious damaging consequences and is a prominent feature in individuals suffering from neurodegenerative diseases. Individuals diagnosed with Parkinson’s have debilitating symptoms including bradykinesia (slowness of movement) and arrests. Approximately 1 million people in the US are diagnosed with Parkinson’s disease and 90,000 individuals are diagnosed annually. There are many current treatments, but there is not success yet in finding a cure to the disease. Deep brain stimulation (DBS) has been used to stimulate dopaminergic neurons in the brain, but its effectiveness decreases within 5-10 years. While there are drugs like levodopa developed to slow the progression of the disease, the therapeutic window narrows over time as well. The true root of the issue remains unclear.
The Renewal
In an attempt to solve this dilemma, we can return back to the birth of the dopamine neuron. A promising approach in the study of neurological diseases is known as regenerative medicine. This approach might involve replacing a patient’s dead neurons with lab-developed (in-vitro) neurons. The process would require stem cells which, when cultured with the necessary transcription factors, can specialize into mDA neurons that could function as a replacement for the patient.
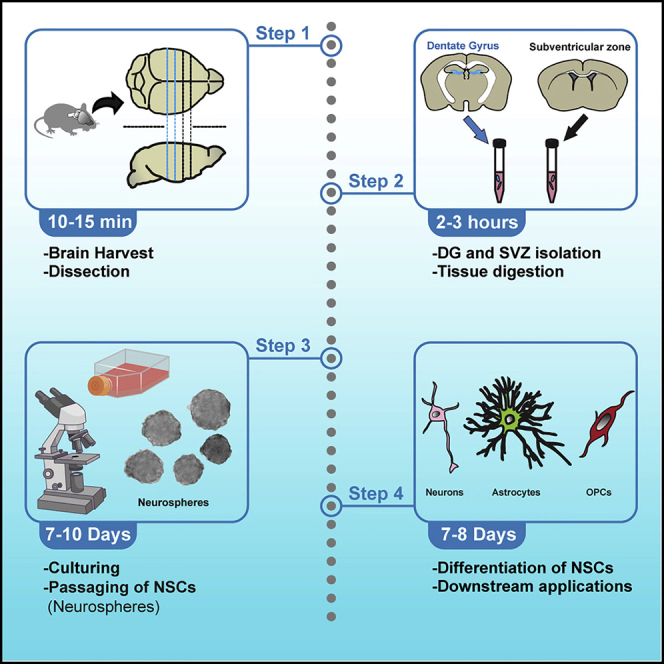
If this process can be accomplished successfully, there is potential for restoring the dopaminergic pathway and regaining some lost motor function. However, many challenges remain in developing stem cell therapies. For example, if differentiated from embryonic stem cells, there is risk in developing tumors from unregulated growth. Additionally, if donated from a different individual, the stem cells may trigger an immune response in the patient. The stem cells may be perceived as foreign from the patient’s immune system—as a result efforts are made to ensure close matching between patient and donor tissues. Even when all these issues are addressed, ensuring that this form of treatment is scalable and broad enough to treat all patients suffering with Parkinson’s disease is a momentous task. Despite these obstacles however, there is potential for harnessing the regenerative power of stem cells to confront this neurodegenerative disease.
Acknowledgements
I would like to thank Nancy Hernandez Villegas, PHD candidate at the Schekman Lab and the BSJ editorial staff for generously reviewing my article for accuracy.
References
- Blandini, F., Nappi, G., Tassorelli, C., & Martignoni, E. (2000). Functional changes of the basal ganglia circuitry in Parkinson’s disease. Progress in neurobiology, 62(1), 63–88. https://doi.org/10.1016/s0301-0082(99)00067-2
- Calabresi, P., Mechelli, A., Natale, G. et al. Alpha-synuclein in Parkinson’s disease and other synucleinopathies: from overt neurodegeneration back to early synaptic dysfunction. Cell Death Dis 14, 176 (2023). https://doi.org/10.1038/s41419-023-05672-9euro
- Lanciego, J. L., Luquin, N., & Obeso, J. A. (2012). Functional neuroanatomy of the basal ganglia. Cold Spring Harbor perspectives in medicine, 2(12), a009621. https://doi.org/10.1101/cshperspect.a009621rt
- Wang, M., Ling, K. H., Tan, J. J., & Lu, C. B. (2020). Development and Differentiation of Midbrain Dopaminergic Neuron: From Bench to Bedside. Cells, 9(6), 1489. https://doi.org/10.3390/cells9061489N
- Ahmed, A. K. M. A., Isaksen, T. J., & Yamashita, T. (2021). Protocol for mouse adult neural stem cell isolation and culture. STAR protocols, 2(2), 100522. https://doi.org/10.1016/j.xpro.2021.100522.
Image References
Banner Image: Mallette, Linnaea. Happy Abstract Sunflower Free Stock Photo – Public Domain Pictures, www.publicdomainpictures.net/en/view-image.php?image=316301&picture=happy-abstract-sunflower. Accessed 28 Nov. 2023.
Figure 1: Wang, M., Ling, K. H., Tan, J. J., & Lu, C. B. (2020). Development and Differentiation of Midbrain Dopaminergic Neuron: From Bench to Bedside. Cells, 9(6), 1489. https://doi.org/10.3390/cells9061489N
Figure 2: File:Dopaminergic pathways.svg. (2021, August 14). Wikimedia Commons. Retrieved 02:31, October 27, 2023 fromhttps://commons.wikimedia.org/w/index.php?title=File:Dopaminergic_pathways.svg&oldid=580788549.
Figure 3: Calabresi, P., Mechelli, A., Natale, G. et al. Alpha-synuclein in Parkinson’s disease and other synucleinopathies: from overt neurodegeneration back to early synaptic dysfunction. Cell Death Dis 14, 176 (2023).
Figure 4: Ahmed, A. K. M. A., Isaksen, T. J., & Yamashita, T. (2021). Protocol for mouse adult neural stem cell isolation and culture. STAR protocols, 2(2), 100522. https://doi.org/10.1016/j.xpro.2021.100522