The New Focus on Electric Vehicles
Fossil fuel use, particularly with gas-powered cars, has been the main culprit for causing climate change and the subsequent increase in global temperatures, rise in sea levels, and extreme weather events. Electric vehicles have emerged as one of the solutions to mitigate climate change in wealthy and high populated countries.1 However, the production of their batteries relies heavily on minerals (such as cobalt found on landmines in the Democratic Republic of the Congo).2 These minerals, if not sourced responsibly, can exacerbate environmental degradation and contribute to the very issue they seek to address. Increased demand for batteries for electrical vehicles, along with the increasing global dependency on electronics, has forced manufacturers to look at deep-sea mineral deposits to supply the necessary materials.3
Deep-sea mineral deposits come in different forms, one of which is polymetallic nodules, potato sized mineral conglomerate with high concentrations of nickel, cobalt, manganese, and copper that take millions of years to form at depths between 4000 and 6000 meters below sea level. These nodules are present in large quantities; like at the Clarion-Clipperton Zone (CCZ), an abyssal plain where most mining activities are likely to start operating first due to its flat topography, abundance of polymetallic nodules, and available technology. In the CCZ there are 34 billion wet metric tons of nodules with a commercial value for the contained metals being about 10 trillion US dollars.3, 4 As such, mining companies have started pressuring governments to accept permits for large-scale deep-sea mineral mining operations in order to meet this demand.

The International Seabed Authority (ISA) is the main regulating body for protecting ecosystems and mineral deposits in the seabed outside of national jurisdiction (referred to as ‘the Area’).5 Exploration permits currently allow companies to find mineral deposits and assess potential environmental impacts of future mining operations by using echo-sounding systems mounted on the hulls of research vessels and autonomous underwater vehicles to map the seafloor’s topography.6 Exploitation permits, on the other hand, allow for commercial mining operations to collect the minerals found during the expeditions. Proposed deep-sea mineral mining plans involve subsea trenching, dredging, and gathering mineral deposits from the sea-floor via remotely operated systems. The main deep sea mineral deposits include polymetallic nodules at abyssal plains, polymetallic crusts (mineral deposits consisting mostly of cobalt that are spread over large expanses of the seafloor) at seamounts and ridges, and seafloor massive sulfides (sulfur-rich mineral precipitates) at hydrothermal vents. 3 Scientists and policymakers have challenged these exploits by expressing concerns over the potential negative environmental impacts of deep-sea mining on marine ecosystems especially since it is challenging to effectively regulate mining operations. These disturbances to marine ecosystems can ultimately hinder the Earth’s ability to sequester carbon and cause irreversible damage through climate change and ocean acidification.6
While there are currently no active commercial deep-sea mineral mining operations, the ISA has issued 31 exploration permits for deep-sea mineral deposits. The ISA had announced its intention to transition an exploration permit to an exploitation permit for the first time for the Republic of Nauru in the CCZ as early as July 2023. This was because Nauru triggered the “two-year rule” of the United Nations Convention on the Law of the Sea by submitting a proposal to start deep-sea mining in the CCZ. The “two-year rule” refers to the ISA being required to develop and adopt rules, regulations, and procedures to govern proposed mining activities within two years of a state submitting an application for an exploitation permit. If the ISA cannot adopt regulations by the end of the two-year period then it must review the mining proposal. It is heavily implied that the ISA must approve of proposed exploitation permits in the absence of regulations that would conflict with proposed plans or disapproval by other member states. However, the approval process for this exploitation permit was stopped in September 2021 after members of the International Union for Conservation of Nature (IUCN) Congress voted overwhelmingly in favor of a moratorium for deep-sea mineral mining. Members in support of a moratorium cited the fact that the ISA did not have comprehensive regulations to effectively protect marine ecosystems and mineral deposits during mining operations.7
Extracting the Minerals
Polymetallic nodules are the primary focus of mining operations because they are easier to collect. Proposed projects plan to pick or scoop up polymetallic nodules and vacuum up sediment layers. The minerals are then transported to the surface vessel via a vertical transport system, such as a pipe. From here, the minerals are separated from any sediment using hydraulic jets and transferred to transport barges. The wastewater containing suspended sediment and mineral particulates is discharged from the vessel at the sea surface or in another pipe to be released at depth.3 This proposed process is incredibly labor-intensive and presents opportunities for significant disturbances to a currently pristine natural ecosystem.

Environmental Concerns
Deep-sea ecosystems are ecologically crucial, and cannot be reduced to a simple monetary value. One of the most important services these ecosystems provide is sequestering a substantial portion of atmospheric carbon dioxide. Currently, “there are about 37,700 gigatons of carbon in organic and inorganic forms in the deep pelagic and 6 billion gigatons of carbon captured in deep-sea sediment.” 8 This carbon is sequestered and stored in the sea-floor sediment so mining operations that disturb the ocean floor risk releasing this carbon back into the ocean and the atmosphere. This would ultimately exacerbate the issue of climate change and contribute to its effects including ocean acidification. Ocean acidification is the process in which the pH level of the Earth’s oceans decreases to become more acidic over time due to the reaction of excess carbon dioxide (CO2) emissions with seawater from the ocean (see Figure 3). When the water becomes too acidic, survival rates drop for most organisms since the calcium carbonate shells of organisms like shellfish and corals dissolve and the growth and development of species throughout their life cycles are hindered. With growth and reproduction of organisms disrupted, many species may eventually face extinction.9 By releasing trapped carbon dioxide into the ocean during mining operations, the risk of experiencing the negative effects of ocean acidification increases.

Furthermore, deep-sea mining exploitation activities will disturb the habitats of various organisms and disrupt non-food-chain-related interactions in the deep-sea ecosystems like how organisms find shelter. Polymetallic nodules provide a vital substrate for organisms like sea sponges and corals, and their removal could be detrimental to the survival of these organisms, as well as the rest of the food web on the abyssal seabed.10 As a result, the overall balance and resilience of these ecosystems are jeopardized, potentially leading to a decline in biodiversity and the loss of unique species that have adapted to the extreme conditions of the abyssal seabed.
Disrupting deep-sea ore deposits can be dangerous since they are rich in potentially toxic elements like copper, cadmium, zinc, and lead which can bioaccumulate in higher trophic levels of the food chain. Although at lower concentrations some of these metals can have positive effects for organisms living in the open ocean at higher concentrations they can be toxic. Low temperature and high pressure in deep-sea environments affect metal uptake and toxic effects differently from standard conditions (25° C at one atmosphere of pressure), making it challenging to regulate deep-sea mining based on exposure thresholds established in conventional settings. Metal uptake can occur through gills, body walls, digestive tracts, or ingestion of crushed mineral particles. Organisms’ detoxification processes consume energy and thus their basal metabolic rates are impacted and the energy available for growth, reproduction, and movement is reduced. If growth and reproduction are impacted then the population sizes decrease and species may be vulnerable to extinction. Additionally, organisms cannot always avoid absorbing the toxic metals. For example, molluscs can close their valves to avoid absorbing toxic metals through their gills. However, they can only close their valves for a short period of time before they must open them to breathe the contaminated water.11 This can be particularly dangerous if hazardous mining operations and their ecosystem disturbances become permanent. This presents the urgent question of how mining operations can minimize the disturbance of sediments and properly treat or dispose of sediment-laden water.
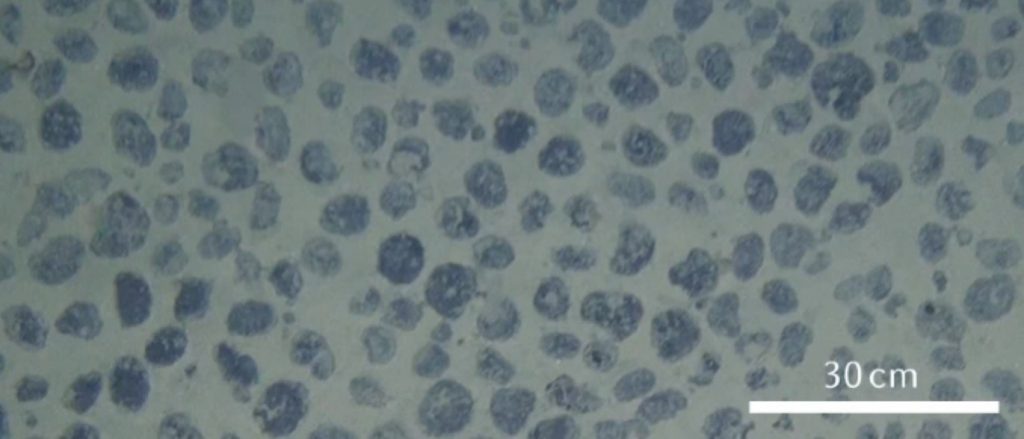
Current Regulations and Legislation
The ISA establishes baseline regulations for issuing permits and reducing the environmental impacts of exploration efforts and potential future mining operations. Currently there are 169 Member States including China and the European Union but notably not the United States of America, since they did not ratify the United Nations Convention of the Law of the Sea.12 In accordance with exploration regulations by the ISA, Member States are required to adopt national laws and regulations for deep-sea mining exploration and developments. The ISA encourages States to consider methods of mitigating the environmental impacts and over-exploitation of deep-sea minerals.
For instance, The Singapore Deep Sea Mining Act of 2015 created specific regulations and procedures for companies to follow and held the country liable for any damage to marine ecosystems. Other examples of regulations include the Pacific Community’s creation of the Pacific Island States Exclusive Economic Zones via a regional legislative and regulatory framework, a regional environmental management framework, and regional scientific research guidelines. This framework helps companies assess the impacts of deep-sea mineral mining activities particularly on an ecosystem’s ability to thrive and offer ecological benefits beyond mineral resources.11 These regulations show the global community’s proactive approach to addressing the environmental and ecological concerns associated with deep-sea mining by assigning liability and requiring ecological assessments. They fail, however, to consider the cumulative impacts of multiple mining activities in the same region, provide specific measures for protecting poorly-understood deep-sea ecosystems, and implement regulations for rehabilitation and remediation to any ecosystems that are harmed by mining operations.

Future Alternatives
As we continue to study the deep-sea ecosystems and the environmental impacts of mineral mining, we can explore alternative sources of fuel and battery minerals for vehicles. There has been recent interest in harvesting cobalt, a mineral used in electric car batteries, from seawater since it is incredibly abundant in the ocean. This is done through passive adsorption technologies, biological processes, and remineralization.13 Another potential way of sourcing minerals needed for electric vehicles is through a continuous electrical pumping membrane process to enrich lithium from seawater.14 Both of these methods of extracting minerals from seawater show promise as they are energy efficient, cost-effective, and have minimal negative environmental impacts. These show that the future of minerals for electrical vehicle batteries may not be from deep-sea mineral deposits.
The future of deep-sea mining is at the forefront of the discussion for mitigating climate change by creating batteries for electric vehicles. The substantial risk of destroying habitats emphasizes the need for a comprehensive assessment of the potential ecological impacts of deep-sea mineral mining before its widespread implementation. To best mitigate the environmental harms of deep-sea mining, more research is needed on alternatives to electric batteries for cars, sources for metals besides deep-sea mineral deposits, and methods to minimize ecosystem disturbances during mining operations.
Acknowledgements
I would like to thank Elisabetta Menini, a Marine Science and Conservation PhD candidate at Duke University, for so generously reviewing my article for accuracy and providing plenty of detailed and thoughtful feedback. Additionally, I would like to thank BSJ Features Editors Luyang Zhang and Aashi Parikh as well as BSJ Managing Editor Varun Upadhyay for their help throughout the writing process.
References
- Wilberforce, El-Hassan, Z., Khatib, F. N., Al Makky, A., Baroutaji, A., Carton, J. G., & Olabi, A. G. (2017). Developments of electric cars and fuel cell hydrogen electric cars. International Journal of Hydrogen Energy, 42(40), 25695–25734. https://doi.org/10.1016/j.ijhydene.2017.07.054
- Calvo, G., & Valero, A. (2022). Strategic mineral resources: Availability and future estimations for the renewable energy sector. Environmental Development, 41, 100640-. https://doi.org/10.1016/j.envdev.2021.100640
- Jones, Daniel O. B., Diva J. Amon, and Abbie S. A. Chapman, ‘Deep-sea mining: processes and impacts’, in Maria Baker, Eva Ramirez-Llodra, and Paul Tyler (eds), Natural Capital and Exploitation of the Deep Ocean (Oxford, 2020; online edn, Oxford Academic, 22 Oct. 2020), https://doi.org/10.1093/oso/9780198841654.003.0005.
- Paulikas, Daina, Katona, Steven, Ilves, Erika, Ali, Saleem H., ‘Life cycle climate change impacts of producing battery metals from land ores versus deep-sea polymetallic nodules,’ Journal of Cleaner Production, Volume 275, 2020, 123822, ISSN 0959-6526, https://doi.org/10.1016/j.jclepro.2020.123822.
- Deep-Sea Mining. IUCN. (2022, July 7). https://www.iucn.org/resources/issues-brief/deep-sea-mining#:~:text=More%20than%201.5%20million%20km,govern%20the%20transition%20to%20exploitation
- Hein, J.R., Koschinsky, A. & Kuhn, T. Deep-ocean polymetallic nodules as a resource for critical materials. Nat Rev Earth Environ 1, 158–169 (2020), https://doi.org/10.1038/s43017-020-0027-0.
- Blanchard, C., Harrould-Kolieb, E., Jones, E., & Taylor, M. L. (2023). The current status of deep-sea mining governance at the International Seabed Authority. Marine Policy, 147, 105396-. https://doi.org/10.1016/j.marpol.2022.105396.
- Thurber, Andrew R., and Amanda N. Netburn, ‘The deep ocean’s link to culture and global processes: nonextractive value of the deep sea’, in Maria Baker, Eva Ramirez-Llodra, and Paul Tyler (eds), Natural Capital and Exploitation of the Deep Ocean (Oxford, 2020; online edn, Oxford Academic, 22 Oct. 2020), https://doi.org/10.1093/oso/9780198841654.003.000.
- Kroeker, K. J., Kordas, R. L., Crim, R., Hendriks, I. E., Ramajo, L., Singh, G. S., … & Gattuso, J. P. (2013). Impacts of ocean acidification on marine organisms: quantifying sensitivities and interaction with warming. Global change biology, 19(6), 1884-1896.
- Stratmann, T., Soetaert, K., Kersken, D. et al. Polymetallic nodules are essential for food-web integrity of a prospective deep-seabed mining area in Pacific abyssal plains. Sci Rep 11, 12238 (2021). https://doi.org/10.1038/s41598-021-91703-4.
- Hauton, Brown, A., Thatje, S., Mestre, N. C., Bebianno, M. J., Martins, I., Bettencourt, R., Canals, M., Sanchez-Vidal, A., Shillito, B., Ravaux, J., Zbinden, M., Duperron, S., Mevenkamp, L., Vanreusel, A., Gambi, C., Dell’Anno, A., Danovaro, R., Gunn, V., & Weaver, P. (2017). Identifying Toxic Impacts of Metals Potentially Released during Deep-Sea Mining—A Synthesis of the Challenges to Quantifying Risk. Frontiers in Marine Science, 4. https://doi.org/10.3389/fmars.2017.00368.
- Jones, Daniel O.B., Durden, Jennifer M., Murphy, Kevin, Kristina M. Gjerde, Aleksandra Gebicka, Ana Colaço, Telmo Morato, Daphne Cuvelier, David S.M. Billett, Existing environmental management approaches relevant to deep-sea mining, Marine Policy, Volume 103, 2019, Pages 172-181, ISSN 0308-597X, https://doi.org/10.1016/j.marpol.2019.01.006.
- Haji, & Slocum, A. H. (2019). An offshore solution to cobalt shortages via adsorption-based harvesting from seawater. Renewable & Sustainable Energy Reviews, 105(C), 301–309. https://doi.org/10.1016/j.rser.2019.01.058
- Li, Li, C., Liu, X., Cao, L., Li, P., Wei, R., Li, X., Guo, D., Huang, K.-W., & Lai, Z. (2021). Continuous electrical pumping membrane process for seawater lithium mining. Energy & Environmental Science, 14(5), 3152–3159. https://doi.org/10.1039/d1ee00354b.
Image References
Figure 1, 2, 4: Hein, J.R., Koschinsky, A. & Kuhn, T. Deep-ocean polymetallic nodules as a resource for critical materials. Nat Rev Earth Environ 1, 158–169 (2020), https://doi.org/10.1038/s43017-020-0027-0.
Figure 3: Mbuhain. The Process of Ocean Acidification. 1 June 2023. https://upload.wikimedia.org/wikipedia/commons/5/53/Ocean_Acidification.jpg?20230601223231.
Figure 5: Jones, Daniel O. B., Diva J. Amon, and Abbie S. A. Chapman, ‘Deep-sea mining: processes and impacts’, in Maria Baker, Eva Ramirez-Llodra, and Paul Tyler (eds), Natural Capital and Exploitation of the Deep Ocean (Oxford, 2020; online edn, Oxford Academic, 22 Oct. 2020), https://doi.org/10.1093/oso/9780198841654.003.0005.