The Milky Way galaxy is fascinating, and not only because it houses the only intelligent life we know of. How old is our Galaxy, and when did Milky Way-like galaxies start to form? The answer to how we got here may be written in the cosmos, in galaxies that mirror our own and perhaps carry the same potential.
One hundred million years after the Big Bang, the structure of the Universe began to take shape from a rapidly expanding sea of gas and dust. This state of matter is referred to as a “particle soup,” consisting of quarks and leptons at 4 trillion degrees Celsius (approximately 250,000 times hotter than the core of the Sun).1 Several hundred thousand years later, the first atoms formed from these initial building blocks, filling the cooling Universe with hydrogen and helium gas. Approximately 830 million years after the Big Bang, gravity began to draw this matter together, gradually forming a cosmic web ripe for galaxy formation (Figure 1). At concentrated regions of the cosmic web, clouds of gas gravitationally contracted, forming dense spots that would later evolve into the galaxies we observe today. Matter collapsed further over billions of years within these dense spots, forming the small-scale structure of the Universe, including the first stars and eventually, planets. The density profile of a galaxy determines the orbital motions of stars, gas, and dust which give the galaxy its unique shape.
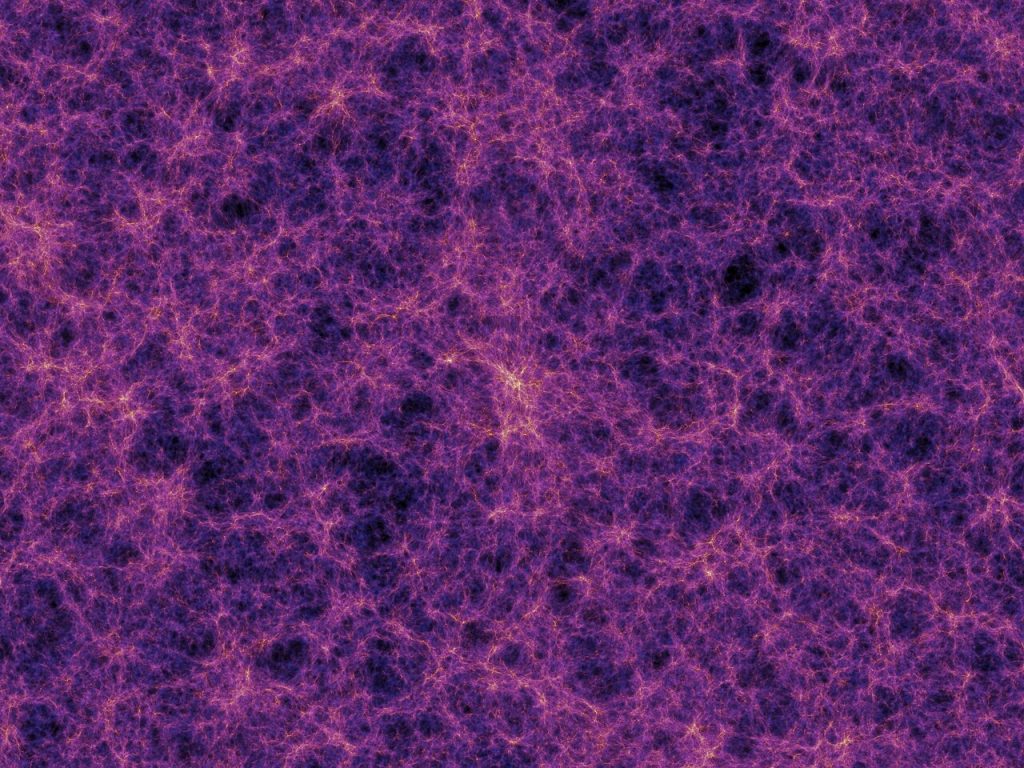
What about our galaxy?
Observations of the oldest stars in the Milky Way revealed their age, confirming that the Galaxy began to form approximately 13 billion years ago.2 For scale, the age of the Universe is roughly 13.7 billion years, meaning galaxies like the Milky Way have been evolving (at least from a primitive state) for the vast majority of the Universe’s existence. Scientists are particularly interested in studying the evolution of the Milky Way and galaxies with similar characteristics because their unique properties eventually led to the formation of life on Earth. While intelligent life cannot be solely attributed to galaxy evolution, studying the properties of the Milky Way is crucial to understanding how stars like our Sun are born and create habitable conditions for planets.
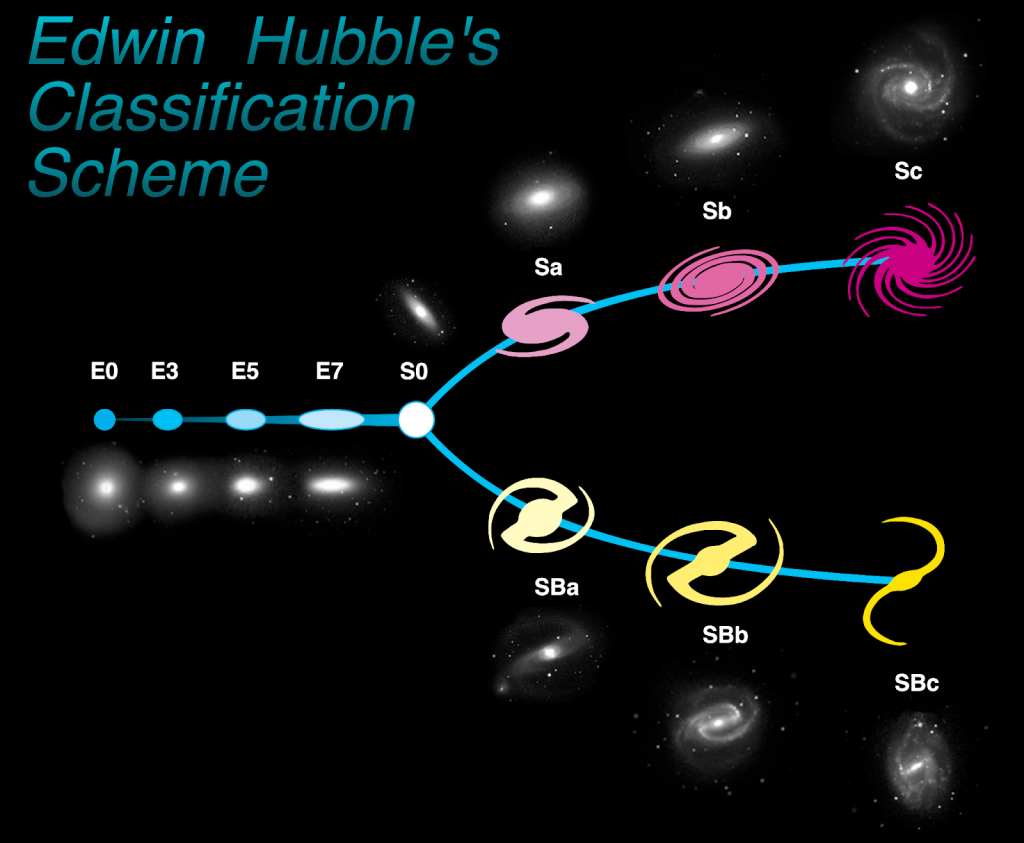
There are at least 2 trillion galaxies in the Universe, each one unique in size and structure.3 In 1926, Edwin Hubble developed the Hubble tuning fork scheme (Figure 2) to classify galaxies based on their morphological features (i.e., whether the structure includes a galactic bar around the center and whether it appears to be spiral, elliptical, or somewhere in between). Hubble’s diagram is a simplified model, but the tuning fork is still used as the foundation for galaxy classification processes today. However, the field is rife with nuance, and consensus in identifying visual features is always evolving. For example, a recent study from the Chinese Academy of Sciences suggests that the Milky Way galaxy, previously thought to have four spiral arms, actually consists of two central arms interspersed with several other fragmented arms.4 These disrupted arms are thought to be caused by interactions with other galaxies in the distant history of the Milky Way.
During the age when the first galaxies began to form, the Universe was smaller and denser than its current state of continued expansion. Therefore, it was much more likely for galaxies to interact with each other, each attracted by the gravity of the other. While this may seem catastrophic, stars are relatively sparse compared to the full volume of a galaxy; hence the chances of star collisions in these interactions are extremely low. Furthermore, only a small fraction of a galaxy consists of “normal” atomic matter. The majority of a galaxy like the Milky Way consists of dark matter, an invisible substance that does not interact with light or the electromagnetic force like atoms do.6
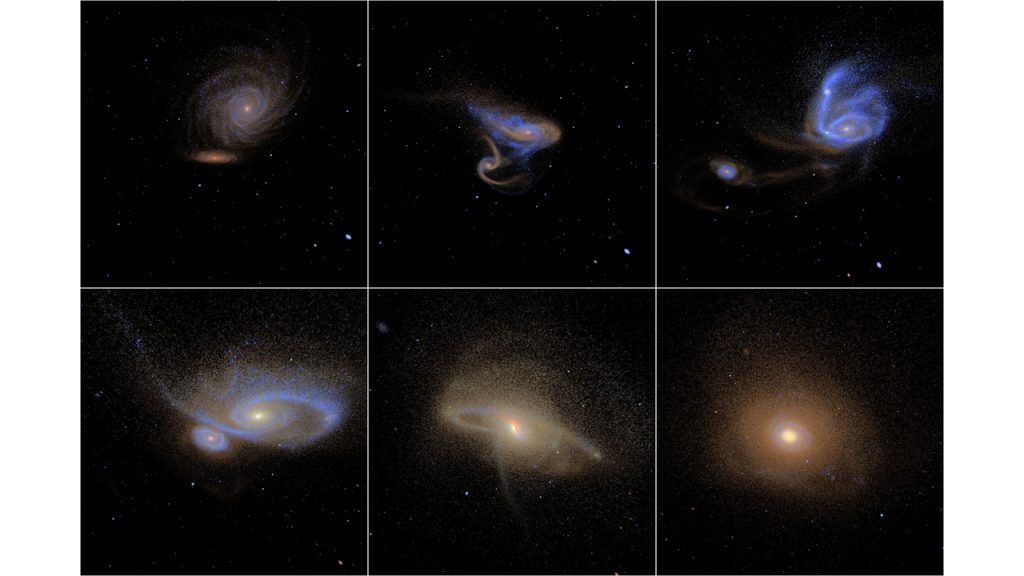
Meaning, while galaxies are still affected by the gravitational force, upon contact, they are not destroyed. Instead, galaxies merge or pass through each other in a “fly-by encounter” if the velocity is high enough. Dark matter plays a role in intergalactic attraction and how the large-scale structure of the galaxy is disrupted. For example, should two spiral disk galaxies merge with one another, the gravitational interactions between the gas and dust in each galaxy wipe away the finer features such as spiral arms, usually resulting in an elliptical galaxy as the final state (Figure 3).
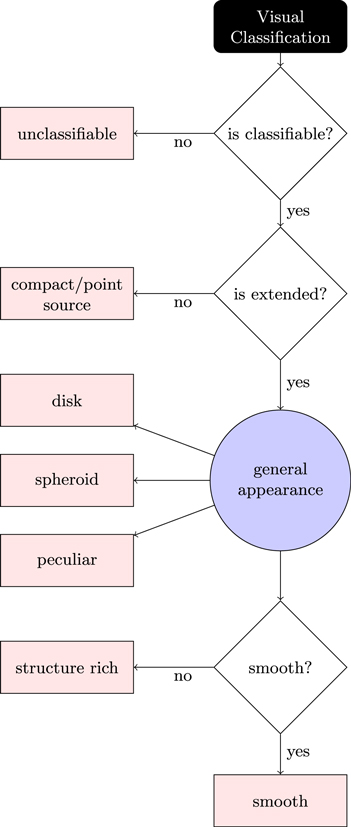
Given the high merger rate in the dense early Universe, researchers believed fully formed spiral galaxies similar to the Milky Way to be unlikely at that time. This was confirmed by results obtained with the Hubble Space Telescope (HST), which observes in near-infrared wavelengths. Hubble’s images from the early Universe show more oblong and elliptical shapes, lacking in intricate features seen in the Milky Way. However, HST has limited capabilities and was only able to observe these regions at redshift z (the fractional change in wavelength) ~ 2.8, which corresponds to roughly 11 billion years into the past.7 Because the speed of light (3.0 x 10^8 m/s) is finite, light takes time to reach HST. The farther away an object is, the more time it will take for that object’s light to be visible to us. Therefore, as telescopes probe more distant galaxies, the images obtained depict the galaxies at earlier and earlier states, allowing scientists to study the Universe at younger intervals.
However, technological advances in observational astronomy are allowing researchers to see further back into the history of the Universe and draw more accurate conclusions about galaxy evolution. In results published in October 2023, a research team from the University of Manchester and the University of Victoria uncovered evidence using the James Webb Space Telescope (JWST) that shows the early Universe was actually rife with Milky Way-like disk galaxies.7 JWST, which launched in December 2021, has higher image resolution than HST and a wavelength filter that can reach up to the mid-infrared wavelengths. Thus, JWST can probe the same regions as HST, but up to z ~ 9 (13.1 billion years into the past), meaning the images are more clear and a more accurate snapshot of the time period. A side-by-side comparison between HST and JWST images of 13 galaxies (Figure 5) reveal morphological features shown more clearly in the JWST images where HST was sometimes only able to capture the galactic center. Each column in Figure 5 shows a galaxy through one of ten different filters (i.e. in different wavelengths of light that correspond to different colors).
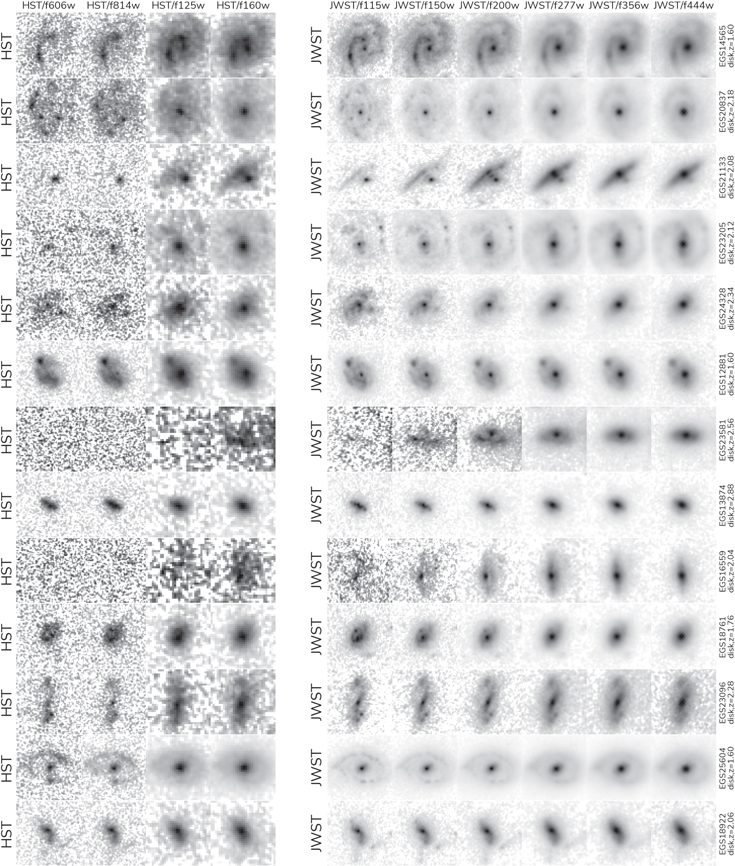
The JWST study was conducted using a sample of 3956 galaxies at 1.5 < z < 6.5 (between roughly 8.5 to 12.8 billion years ago). Using a classification algorithm called MORFOMETRYKA, these scientists were able to study the morphological features of young galaxies at an unprecedented volume. Within the sample, they identified 1672 disk galaxies, 553 spherical, 1100 peculiars, 428 ambiguous sources, 55 point sources, and 148 unclassifiable sources.7 With disk galaxies at 42% abundance, these results show that a vast amount of galaxies with detailed structure similar to the Milky Way were able to survive in the denser conditions of the early Universe.
This research suggests that our current knowledge of galaxy evolution and the greater evolution of the Universe must be re-examined. The huge presence of fine galaxy features signals that the structure of the Universe developed more quickly than previously thought, and also raises a host of new questions. The regularity of fully formed disk galaxies with detailed structure shortly after the beginning of the Universe suggests “that most stars exist and form within these galaxies.”8 How common were stars like our Sun in the early Universe? Did life begin to develop during this stage as well?
Our view of the Universe is only as accurate as technology allows, for we only truly know what we are able to detect. Astrophysics is constantly evolving. The Universe is riddled with Milky Way galaxies and has been for far longer than expected. What was considered fact by the Hubble telescope has now been rendered an outdated view of the Universe as JWST surveys the cosmos.
Acknowledgements
Thank you to Natasha Abrams, a graduate student in the Astrophysics department for reviewing my article and for being a wonderful teacher. I’d also like to thank my Features editors Luyang Zhang and Aashi Parikh for their patience and guidance this semester.
References
- PHENIX Collaboration. (2018). Creation of quark–gluon plasma droplets with three distinct geometries. Nature Physics, 15(3), 214–220. https://doi.org/10.1038/s41567-018-0360-0
- Xiang, M., & Rix, H. W. (2022). A time-resolved picture of our Milky Way’s early formation history. Nature, 603(7902), 599-603.
- Conselice, C. J., Wilkinson, A., Duncan, K., & Mortlock, A. (2016). The evolution of galaxy number density at z< 8 and its implications. The Astrophysical Journal, 830(2), 83.
- Xu, Y., Hao, C. J., Liu, D. J., Lin, Z. H., Bian, S. B., Hou, L. G., … & Li, Y. J. (2023). What Does the Milky Way Look Like?. The Astrophysical Journal, 947(2), 54.
- Conselice, C. J. (2014). The evolution of galaxy structure over cosmic time. Annual Review of Astronomy and Astrophysics, 52, 291-337.
- Ou, X., Eilers, A. C., Necib, L., & Frebel, A. (2023). The dark matter profile of the Milky Way inferred from its circular velocity curve. arXiv preprint arXiv:2303.12838.
- Ferreira, L., Conselice, C. J., Sazonova, E., Ferrari, F., Caruana, J., Tohill, C. B., … & Wilkins, S. M. (2023). The JWST Hubble Sequence: The Rest-frame Optical Evolution of Galaxy Structure at 1.5< z< 6.5. The Astrophysical Journal, 955(2), 94.
- University of Manchester. (2023, September 22). Astronomers find abundance of Milky Way-like Galaxies in early Universe, rewriting cosmic evolution theories. ScienceDaily. Retrieved January 14, 2024 from www.sciencedaily.com/releases/2023/09/230922110758.htm
Image References
Figure 1: Volker Springel (Max Planck Institute for Astrophysics) et al. (March 10th, 2020)
Figure 2: Cosmogoblin, CC0, via Wikimedia Commons (August 14th, 2022)
Figure 3: P. Jonsson (Harvard-Smithsonian Center for Astrophysics), G. Novak (Princeton University), and T.J. Cox (Carnegie Observatories, Pasadena, Calif.) (October 27, 2011)
Figure 4: Reprinted from “The JWST Hubble Sequence: The Rest-frame Optical Evolution of Galaxy Structure at 1.5<z<6.5,” by L. Ferreira, 2023, Astrophysical Journal, Volume 955, pg. 3. Copyright 2023 by the authors.
Figure 5: Reprinted from “The JWST Hubble Sequence: The Rest-frame Optical Evolution of Galaxy Structure at 1.5<z<6.5,” by L. Ferreira, 2023, Astrophysical Journal, Volume 955, pg. 13. Copyright 2023 by the authors.