A Serendipitous Discovery: “Blut-Hirn-Schranke”
In the late nineteenth century, German scientist Paul Ehrlich was on a mission to find a cure for “sleeping sickness”— a severe parasitic disease that causes disturbances in sleep patterns and hypoxemia. To measure resting organ oxygen consumption, Ehrlich used tracer dyes that changed color when oxidized.1 However, after many repeated experiments, Ehrlich realized that his intravenous dyes stained every tissue except the brain. It became apparent that some impenetrable barrier surrounded the brain, preventing certain compounds from entering the cerebral space. This realization only amplified the mystique surrounding neurobiology and the complex physiology of the brain.
A few years later, a term for this membrane was coined in Germany: “Blut-Hirn-Schranke,” or the Blood-Brain Barrier (BBB).2
The widespread implications of Ehrlich’s discovery would materialize in 1914 when salvarsan (a drug for neurotic syphilis) could not enter the brain due to the BBB. Eventually, a pattern emerged: The vast majority of drugs and medicine were unable to infiltrate the brain.
In 2024, the BBB still poses a threat to targeted drug therapies, but translational scientists have found ways to harness the unique chemistry of the brain to design medicines capable of permeating the barrier.
What is the Blood-Brain Barrier?
The BBB is a selective, semi-permeable membrane that only permits certain substances to pass between the bloodstream and the interstitium (the fluid-filled connective region between neural cells and blood vessels).
The BBB serves many functions, including supplying the brain with nutrients, regulating communications within the nervous system, and maintaining neuronal homeostasis.3 Most notably, though, the BBB is the brain’s ultimate protector and regulates what molecules enter the brain from the bloodstream. While the BBB permits entry to biologically necessary molecules, it also filters harmful and toxic compounds out of the brain through physiological and metabolic protective measures.
The cells of the BBB are physiologically designed to limit molecular transport. For one, the endothelial cells lining the brain’s blood vessels have no perforations and are lined with tight junctions. These junctions are protein complexes that create a tight seal between cells to restrict diffusion and vesicular transport. This is in stark contrast to the porous endothelial cells of other organs’ blood vessels. Additionally, the endothelial cells within the brain are composed of lipid-rich cell membranes interspersed with efflux transporters; these are membrane-bound proteins that remove waste and flagged substances out of cells. Efflux transporters make it difficult for most substances to pass into the brain.
With all of these blocking mechanisms, a question arises: What types of molecules can surpass this seemingly impenetrable membrane without the use of transporters? In short—molecules that are lipid soluble and possess a very small molecular weight. These permeability constraints prevent the majority of molecules from passing through the BBB. While chemicals like oxygen, carbon dioxide, and electrolytes can easily enter the brain, the majority of therapeutic drugs are inhibited by the BBB. This is because many pharmaceuticals are too big and polar to cross the barrier. According to William Pardridge of the UCLA Department of Medicine, it is estimated that “greater than 90% of all small-molecule drugs and close to 100% of all larger therapeutics cannot overcome the BBB.”4
However, as modern scientists learn more about the brain’s filtration system, new and crafty delivery systems have been chemically designed to assist drugs in bypassing the BBB.
Bypassing Efflux Transporters: Pluronic
Many recent innovations in the drug development sphere have been designed around circumventing the BBB.
Such innovations include Pluronic block copolymers—agents that are co-administered with drug delivery and inhibit the efflux transporters of the BBB.5 Recall that efflux transporters are embedded proteins that pump potentially harmful substances, like toxins or drugs, out of the brain. A key efflux transporter present within the endothelial cells of the BBB is the P-glycoprotein (Pgp) transporter. Pgp is an ATP-dependent transporter that is responsible for exporting the majority of rejected drugs from the brain back into the blood.6 However, Pluronic block copolymers are shown to be effective at hindering Pgp activity.
Pluronic is composed of both a hydrophobic region (propylene oxide) and a hydrophilic region (ethylene oxide). This hydrophilic-lipophilic structure allows Pluronic to permeate cells of the BBB and temporarily diminish mitochondrial output. This in turn disrupts the energy supply needed for ATP-dependent efflux transporters like Pgp to function.
Pluronic block copolymers are an effective way to assist a drug in surpassing the BBB without having to alter the chemistry or formula of the drug altogether. However, there are a couple of drawbacks to this technology. For one, although Pluronic can enhance the transport of many drugs, there are certain types of drugs that do not benefit from the copolymer. For example, drugs with highly hydrophilic properties cannot permeate the cell membrane even with assistance from Pluronic. Additionally, there are some concerns about the toxicity of Pluronic since it contributes to ATP depletion within the brain. However, this effect is shown to be temporary and does not compromise cellular functions in the long term.
A Large Molecule Solution: Receptor-Mediated Delivery
Given that Pluronic is primarily effective in enhancing the transport of small molecules, how would large biomacromolecules (e.g. DNA for gene therapy) be transported across the BBB?
This is where capitalizing on a natural biochemical phenomenon comes into play. Transcytosis, or the receptor-mediated transport of macromolecules, allows certain peptides (i.e. small proteins) to cross the BBB. Each of these peptides (often called homing peptides) binds to specific receptors on brain endothelial cells and is then engulfed by the cells.5 By identifying these unique peptides and combining them with drug carriers (such as nanoparticles, polymer micelles, or liposomes), homing peptides can be used to direct therapeutic agents through the BBB and release them inside the brain..
Altering the Nature of Proteins: Artificial Hydrophobization
Another solution to crossing the BBB requires modification of the drugs themselves to become more transportable through a process called artificial hydrophobization.
Artificial hydrophobization involves conjugating fatty acid residues to drug proteins. This enhances a drug’s ability to bind to lipid membranes within the brain and infiltrate the cell. Studies show that this technique increases the concentration of drugs that penetrate the brain when compared to unmodified proteins.5 Additionally, hydrophobization might also reduce immunogenicity (or the unnecessary provocation of the immune system) since fatty acid chains can mask marker regions on the drug that would normally be recognized and attacked by immune cells.
An Non-Invasive Approach: Ultrasound Waves
One of the most recent breakthroughs in neurotechnology has been the use of Focused Ultrasound (FUS) in conjunction with microbubbles to induce localized openings within the BBB.
Microbubbles are tiny, gas-filled bubbles that can be safely injected into the bloodstream surrounding the brain. After injection, low-frequency ultrasound waves are directed at specific regions of the brain, causing these microbubbles to vibrate and oscillate. The disruption and movement of these bubbles exert a mechanical pressure on the endothelial cells of the BBB, temporarily loosening the tight junctions.7 As pores begin to form in between endothelial cells, larger drugs can pass into specific parts of the brain.
Using FUS and microbubble technology is both a non-invasive and reversible mechanism that shows promise in expanding the scope of drugs able to penetrate the BBB. Another bonus is that FUS-mediated openings can be easily monitored through MRI, allowing for additional safety measures.
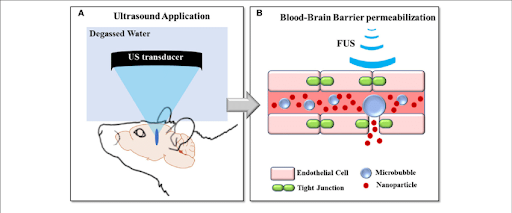
Closing Thoughts
In summary, the amazingly protective and somewhat stubborn nature of the BBB allows biologically necessary molecules to enter the brain while filtering out seemingly harmful, unknown substances. Unfortunately, some molecules that the brain deems as harmful include life-saving medicines and therapeutic interventions. As neuroscience innovations have proliferated over this past century, a variety of techniques have the potential to overcome the hurdle posed by the BBB. With technologies like block polymers and targeted ultrasound waves, the new generation of drug delivery methods will revolutionize the success of brain disease treatment, whether cancerous, neurodegenerative, or infectious.
Turning back to Paul Ehrlich and his serendipitous discovery of the BBB in the 19th century, a question arises: Did he ever fulfill his original goal and find a cure for sleeping sickness?
While not directly, his innovations paved the way for the creation of Fexinidazole—one of the first sleeping sickness drugs capable of passing the BBB without inducing neurotoxicity. In fact, Fexinidazole was designed to be extremely small and lipophilic—and thus capable of entering the brain through simple diffusion. Although it took over 40 years of R&D for this drug to be compatible with the unique biochemistry of the brain, Fexinidazole has been approved as an effective cure for sleeping sickness by the World Health Organization as of 2018. Therefore, while Paul Ehrlich did not find a direct cure for sleeping sickness, his discovery of the BBB catalyzed the creation of the only life-saving drug for the disease. This goes to show that by understanding the unique physiology of the BBB, extremely fatal brain diseases can become treatable.
Acknowledgements
I would like to thank Cholopat Varongchayakul, a chemistry Ph.D. candidate at the University of California, Berkeley, for reviewing this article and offering insightful feedback.
References
- Pardridge, W. M. (2022). A Historical Review of Brain Drug Delivery. Pharmaceutics, 14(6), 1283. https://doi.org/10.3390/pharmaceutics14061283
- Jiang, Y. (2017). Guardian of the brain: The blood-brain barrier. Science in School, (42), 18–22. Retrieved from https://www.scienceinschool.org/article/2017/guardian-brain-blood-brain-barrier/
- Wu, D., Chen, Q., Chen, X., & et al. (2023). The blood–brain barrier: Structure, regulation and drug delivery. Signal Transduction and Targeted Therapy, 8(217). https://doi.org/10.1038/s41392-023-01481-w
- Dotiwala, A. K., McCausland, C., & Samra, N. S. (2023). Anatomy, head and neck: Blood-brain barrier. In StatPearls [Internet]. Treasure Island, FL: StatPearls Publishing. Retrieved from https://www.ncbi.nlm.nih.gov/books/NBK519556/
- Kabanov, A. V., & Batrakova, E. V. (2004). New technologies for drug delivery across the blood brain barrier. Current pharmaceutical design, 10(12), 1355–1363. https://doi.org/10.2174/1381612043384826
- Batrakova, E. V., Li, S., Vinogradov, S. V., Alakhov, V. Y., Miller, D. W., & Kabanov, A. V. (2001). Mechanism of pluronic effect on P-glycoprotein efflux system in blood-brain barrier: contributions of energy depletion and membrane fluidization. The Journal of pharmacology and experimental therapeutics, 299(2), 483–493.
- Konofagou, E. E., Tung, Y. S., Choi, J., Deffieux, T., Baseri, B., & Vlachos, F. (2012). Ultrasound-induced blood-brain barrier opening. Current pharmaceutical biotechnology, 13(7), 1332–1345. https://doi.org/10.2174/138920112800624364
Image References
- Banner Image: Guerin, C. (2014). Blood-brain barrier, light micrograph [Micrograph]. Science Photo Library. https://www.sciencephoto.com/media/306645/view
- Figure 1: Nascari, D. & Sved, A. (2019). Blood brain barrier [PNG image of capillaries]. Wikimedia Commons. https://commons.wikimedia.org/wiki/File:Blood_brain_barrier.png
- Figure 2: Fatima, S., Mohammad, T., Jairajpuri, D., Rehman, M., Hussain, A., Samim, M., Ahmad, F., & Alajmi, M. (2019). Identification and evaluation of glutathione conjugate gamma-L-glutamyl-L-cysteine for improved drug delivery to the brain. Journal of Biomolecular Structure & Dynamics, 37(1), 1-15. https://doi.org/10.1080/07391102.2019.1664937
- Figure 3: Conti, A., Kamimura, H., Novell, A., Duggento, A., & Toschi, N. (2020). Magnetic resonance methods for focused ultrasound-induced blood-brain barrier opening. Frontiers in Physics, 8, 547674. https://doi.org/10.3389/fphy.2020.547674